TL;DR
White blood cells (WBCs), also known as leukocytes, are the body’s defense system cells. They play a crucial role in the immune response, protecting the body from:
- Infections: Bacteria, viruses, parasites, and other pathogens.
- Foreign substances: Dust, pollen, and other foreign particles.
- Abnormal cells: Cancer cells and pre-cancerous cells.
Types of White Blood Cells ▾
- Neutrophils: Phagocytize bacteria and other pathogens.
- Monocytes: Differentiate into macrophages that phagocytize larger particles and present antigens to other immune cells.
- Lymphocytes: Divided into B and T cells, responsible for the adaptive immune response:
- B cells: Produce antibodies to neutralize pathogens.
- T cells: Orchestrate the immune response by activating other immune cells and directly killing infected or tumor cells.
- Eosinophils: Involved in allergic reactions and parasitic infections.
- Basophils: Role is not fully understood, but thought to be involved in allergic reactions, wound healing, and defense against parasites.
White Blood Cell Role in Inflammation ▾
White blood cells (WBCs) play a critical role in inflammation, the body’s response to injury, infection, or irritation. They work together to:
- Identify and eliminate invaders through phagocytosis and other mechanisms.
- Initiate and coordinate the immune response by releasing signaling molecules that attract and activate other immune cells.
- Promote tissue repair by clearing debris and promoting new tissue growth.
Benign and Malignant White Blood Cells Disorders ▾
- Benign White Blood Cell Disorders
- Leukocytosis
- Eosinophilia
- Basophilia
- Malignant White Blood Cell Disorders
*Click ▾ for more information
Definition of White Blood Cell (WBC)
White blood cells (WBCs) are the body’s defense system against infection. They are part of the immune system and are constantly circulating throughout the bloodstream and lymphatic system, patrolling for invaders like bacteria, viruses, and fungi. White blood cells (WBCs) are also called leukocytes (from the Greek words “leukos” meaning white and “cyte” meaning cell). There are a few types of white blood cells namely neutrophils, eosinophils, basophils, monocytes and lymphocytes.
Hematopoiesis
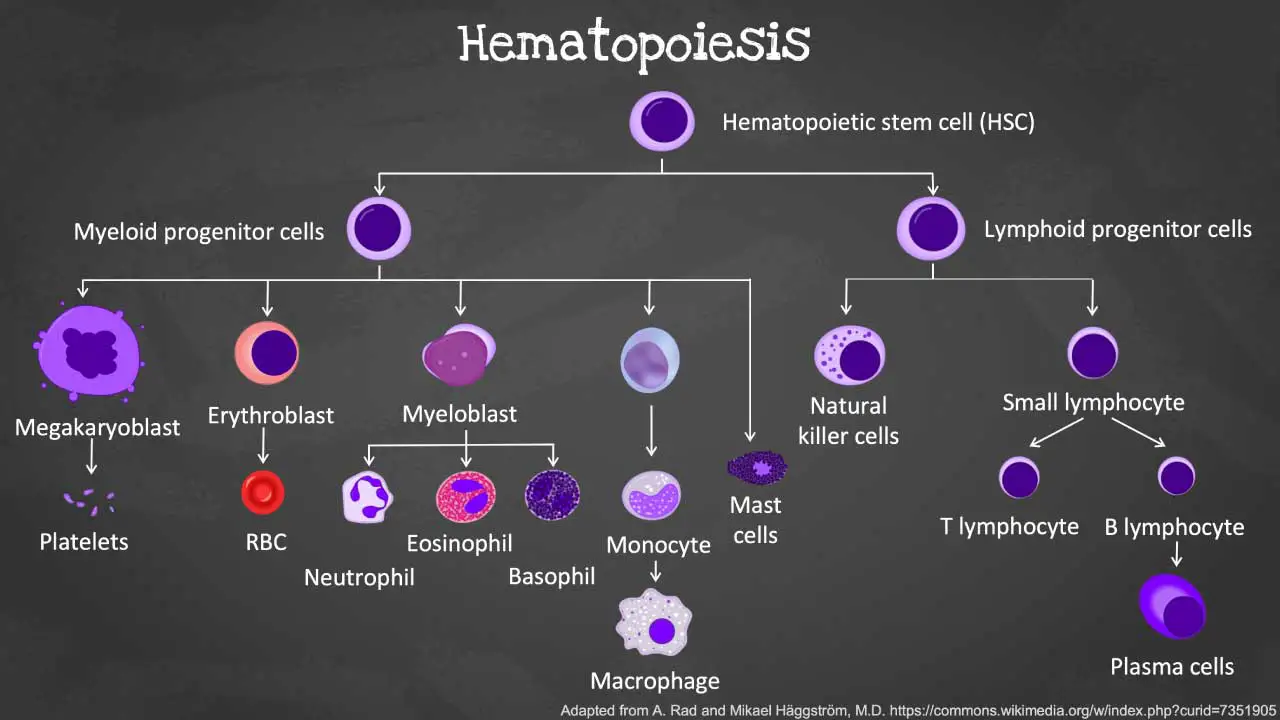
Hematopoiesis is the remarkable process by which our bodies continuously produce a vast array of specialized blood cells including the different types of white blood cells (WBCs). This complex journey begins with a single cell type and unfolds in a series of precisely orchestrated steps:
Hematopoietic Stem Cells (HSCs)
The foundation of blood cell production lies in HSCs, residing primarily in the bone marrow of adults and the fetal liver during development. These self-renewing cells have the unique ability to divide and produce daughter cells with two potential fates:
- Remain as HSCs: This ensures a continuous supply of stem cells for long-term blood cell production.
- Differentiate into common myeloid progenitors (CMPs) or common lymphoid progenitors (CLPs): These are the first committed steps toward specific blood cell lineages.
Multipotent Progenitor Cells
Through a tightly regulated process, HSCs undergo differentiation into multipotent progenitor cells. These progenitor cells still have the ability to differentiate into multiple lineages but are more committed than HSCs and can give rise to:
- Lymphoid progenitors: Precursors for lymphocytes (T and B cells, NK cells), a subtype of white blood cells (WBCs).
- Myeloid progenitors: Precursors for all other mature blood cells like RBCs, granulocytes (neutrophils, eosinophils, basophils), monocytes, macrophages, dendritic cells, and platelets.
Common myeloid progenitors (CMPs)
These progenitors give rise to various myeloid cells, including:
- Granulocyte-macrophage progenitors (GMPs): Further differentiate into:
- Neutrophil progenitors: Develop into neutrophils, the most abundant white blood cells (WBCs), responsible for phagocytosing bacteria and fungi.
- Eosinophil progenitors: Mature into eosinophils, a type of white blood cell (WBC) involved in allergic reactions and parasite defense.
- Basophil progenitors: Give rise to basophils, a type of white blood cell (WBC) which release histamine and other mediators during allergic reactions.
- Monocyte-macrophage progenitors (MMoPs): Differentiate into:
- Monocytes: These type of white blood cells (WBCs) circulate in the blood and migrate to tissues, where they mature into macrophages, responsible for phagocytosis and antigen presentation.
- Dendritic cells (DCs): Specialized antigen-presenting white blood cell (WBC) crucial for initiating the adaptive immune response.
- Megakaryocyte-erythroid progenitors (MEPs): Develop into:
- Megakaryocytes: Large cells that fragment into numerous platelets, essential for blood clotting.
- Erythrocyte progenitors: Mature into red blood cells (RBCs), responsible for oxygen transport throughout the body.
Common Lymphoid Progenitors (CLPs)
These progenitors migrate to the thymus (T cells) or lymphoid organs (B cells and NK cells) for further development and maturation:
- B cell progenitors: Differentiate into B lymphocytes (B cells), which produce antibodies to fight infections.
- T cell progenitors: Migrate to the thymus for further development and maturation into various T lymphocyte (T cell) subtypes, including:
- Helper T cells (Th cells): Orchestrate the immune response by activating other immune cells.
- Cytotoxic T cells (Tc cells): Directly eliminate infected and cancer cells.
- Regulatory T cells (Tregs): Suppress the immune response to prevent autoimmune reactions.
Leukopoiesis
Leukopoiesis is a sub-process of hematopoiesis specifically focused on the formation and development of the different types of white blood cells (WBCs).
Cytokines and their role in leukopoiesis
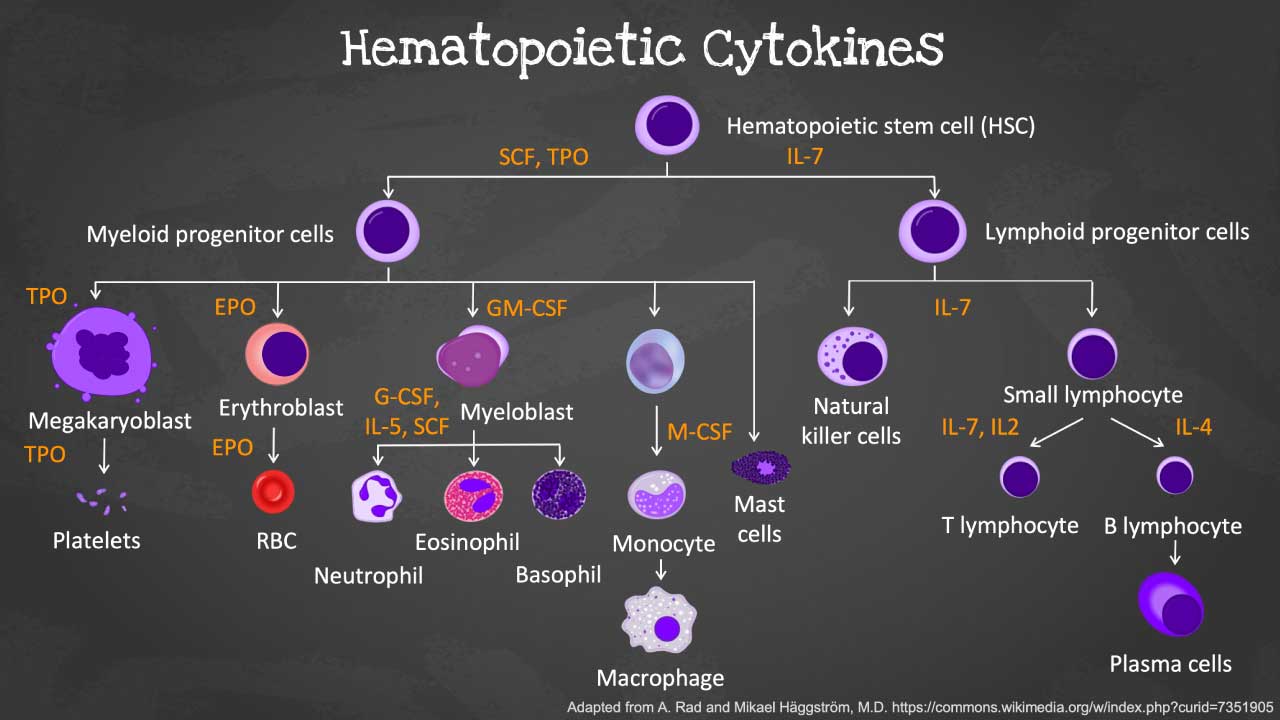
Cytokines are a group of small signaling molecules involved in various cellular communication processes, including regulating the development, activation, and function of immune cells. In the context of leukopoiesis, they play a crucial role in orchestrating the various stages of white blood cell (WBC) development and maturation.
- Stimulate proliferation and differentiation: Cytokines can induce the multiplication and specialization of precursor cells into specific types of white blood cells, such as granulocytes, monocytes, and lymphocytes.
- Promote survival and maturation: They can influence the survival and maturation of developing white blood cells (WBCs), ensuring they reach their functional state.
- Modulate immune response: Various cytokines can influence the activity and function of mature white blood cells WBCs, affecting aspects like migration, activation, and effector functions.
Key cytokines involved in leukopoiesis
- Interleukin-3 (IL-3): Supports the proliferation and differentiation of various blood cell types, including granulocytes, monocytes, and megakaryocyte precursors (platelet precursors).
- Granulocyte-macrophage colony-stimulating factor (GM-CSF): Stimulates the development of granulocytes (neutrophils, eosinophils, basophils) and monocytes.
- Granulocyte colony-stimulating factor (G-CSF): Promotes the proliferation and differentiation of granulocyte precursors, particularly neutrophils.
- Interleukin-6 (IL-6): Stimulates the growth and differentiation of B and T lymphocytes.
- Interleukin-7 (IL-7): Plays a crucial role in the development and survival of T cells.
- Interleukin-11 (IL-11): Supports the development of megakaryocytes and platelet production.
Growth factors and hormones
In addition to cytokines, other signaling molecules also play significant roles in leukopoiesis.
- Growth factors
- Erythropoietin (EPO): While primarily involved in red blood cell production, EPO can also influence granulocyte development.
- Thrombopoietin (TPO): Essential for the production of platelets.
- Stem cell factor (SCF): Supports the self-renewal and survival of hematopoietic stem cells (HSCs), the precursors for all blood cells, including the different types of white blood cells (WBCs).
- Hormones
- Thyroid hormones: Influence the proliferation and maturation of various blood cell types, including some types of white blood cells (WBCs).
- Glucocorticoids (cortisol): Regulate the production of granulocytes and monocytes.
- Sex hormones (estrogen, testosterone): Can influence the production of some immune cells, including some types of white blood cells (WBCs).
Interaction and Complexities
It’s important to note that the specific effects of these molecules depend on various factors, including their concentration, the stage of development they impact, and their interaction with other signaling pathways. They often work in concert, with one cytokine influencing the production or activity of another, creating a complex yet coordinated regulatory network in leukopoiesis.
Granulopoiesis
Granulopoiesis is a sub-process of leukopoiesis, which in turn is a sub-process of hematopoiesis. Here’s a breakdown of the terms:
- Hematopoiesis: The overall process by which all blood cells are formed, including red blood cells (RBCs), white blood cells (WBCs), and platelets.
- Leukopoiesis: The specific process within hematopoiesis that focuses on the formation and development of the different types of white blood cells (WBCs).
- Granulopoiesis: A further specialized process within leukopoiesis that deals specifically with the production and maturation of granulocytes, a specific type of white blood cell.
Therefore, granulopoiesis is the dedicated pathway within leukopoiesis that generates neutrophils, eosinophils, and basophils, collectively known as granulocytes. These specialized type of white blood cell (WBC) are characterized by the presence of granules in their cytoplasm, which contain various enzymes and molecules essential for their defensive functions. Therefore, granulopoiesis can be defined as the development and differentiation of granulocytes from their precursor cells in the bone marrow.
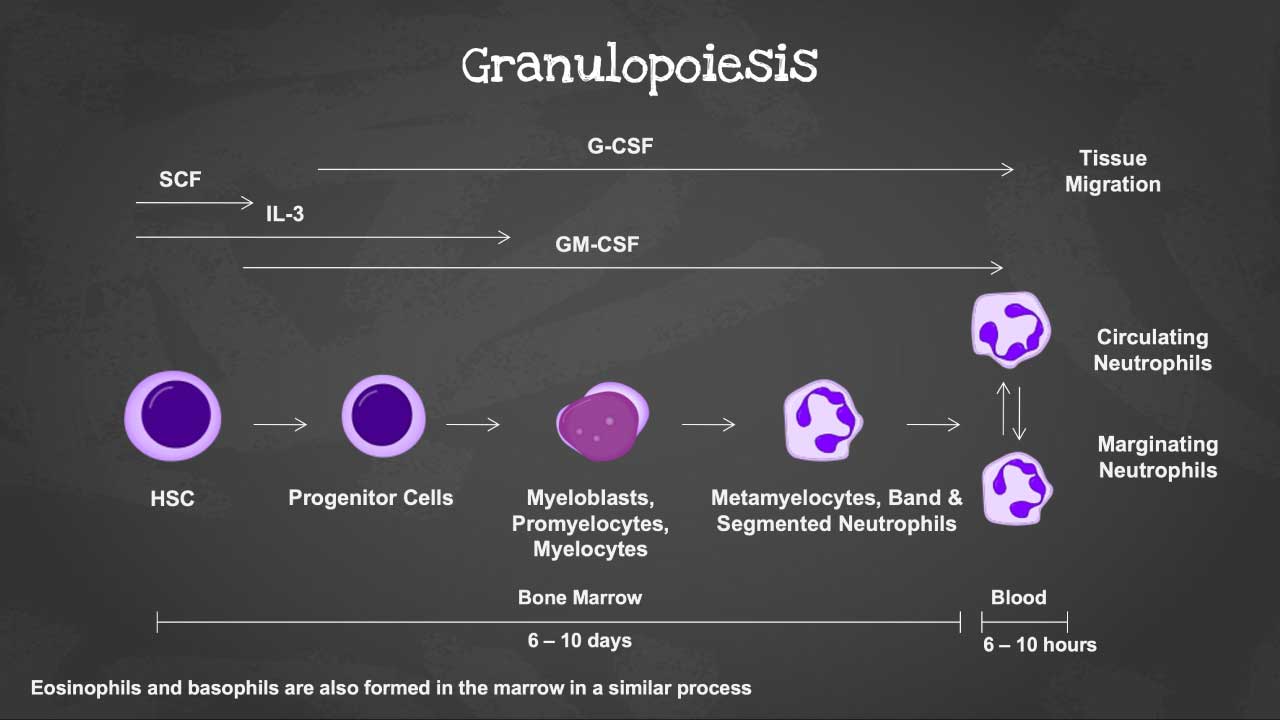
Stages of Granulopoiesis
Stages | Duration (days) | Cytokines | Description and Function |
Myeloblast | 3-4 | GM-CSF, IL-3 | Immature precursor cell, undergoes rapid proliferation. Lacks granules and has a large nucleus with prominent nucleoli. |
Promyelocyte | 2-3 | GM-CSF, IL-3 | Begins developing specific granules in the cytoplasm. Nucleus remains large and prominent. |
Myelocyte | 2-3 | Neutrophils: Granulocyte colony-stimulating factor (G-CSF) Eosinophils: IL-3, IL-5 Basophils: IL-3, Stem Cell Factor (SCF) | Further matures, acquires characteristic granules specific to each type of granulocyte. Nucleus becomes smaller and less prominent. |
Metamyelocyte | 1-2 | Neutrophils: Granulocyte colony-stimulating factor (G-CSF) Eosinophils: IL-3, IL-5 Basophils: IL-3, Stem Cell Factor (SCF) | Undergoes significant nuclear condensation. Cytoplasm becomes more abundant. |
Band form | 1-2 | Neutrophils: Granulocyte colony-stimulating factor (G-CSF) Eosinophils: IL-3, IL-5 Basophils: IL-3, Stem Cell Factor (SCF) | Mature granulocyte with a non-segmented, horseshoe-shaped nucleus. Granules are fully formed and functional. |
Segmented neutrophil /eosinophil /basophil | 0-1 | Final mature granulocyte with a segmented, multi-lobed nucleus. Fully equipped for its specific immune function (phagocytosis, inflammation, etc.). |
Lymphopoiesis
Lymphopoiesis is the specialized process by which the body produces lymphocytes, a crucial type of white blood cell (WBC) responsible for the adaptive immune response. This complex process transforms hematopoietic stem cells (HSCs) into various types of mature lymphocytes with distinct functions in fighting infections and maintaining immune memory.
B Lymphopoiesis
B-lymphocytes, also known as B cells, play a crucial role in the adaptive immune response by producing antibodies – highly specific molecules that can recognize and neutralize pathogens. This intricate process of B cell development, known as B-lymphopoiesis, occurs primarily in the bone marrow.
Stage | Description | Cytokines Involved | Duration (Approximate) |
Pro-B cell | Early B cell precursor arising from common lymphoid progenitors (CLPs). | Interleukin-7 (IL-7), Stem cell factor (SCF) | 3-5 days |
Pre-B cell | Starts rearranging its immunoglobulin heavy and light chain genes to create antigen-specific receptors. | IL-7, Interleukin-2 (IL-2), Interleukin-9 (IL-9) | 7-10 days |
Large Pre-B cell | Undergoes selection in the bone marrow, ensuring the newly rearranged immunoglobulin genes are functional and self-tolerant (don’t recognize self-antigens). | IL-7, B-cell activating factor (BAFF) | 3-5 days |
Small Pre-B cell | Loses its cytoplasmic Ig heavy chain and continues light chain rearrangement. | IL-7 | Variable (days to weeks) |
Immature B cell | Expresses complete immunoglobulin molecules (antibodies) on its surface, specific to a single antigen. | IL-7 | Variable (days to weeks) |
Mature B cell (Naive B cell) | Exits the bone marrow and migrates to lymphoid organs (spleen, lymph nodes). These naive B cells haven’t encountered their specific antigen yet. | B-cell activating factor (BAFF), A proliferation-inducing ligand (APRIL) | – |
Plasma cell | When a naive B cell encounters its specific antigen in the lymphoid organs, it undergoes further differentiation and activation. | Interleukin-6 (IL-6), Transforming growth factor beta (TGF-β) | Variable (days to weeks) |
Additional Notes
- The duration of each stage can vary depending on individual factors and the success of gene rearrangement events.
- Upon activation by their specific antigen and interacting with T helper cells, naive B cells differentiate into plasma cells.
- Plasma cells are specialized B cells focused on antibody production and don’t require further cytokines for their function. They can produce large quantities of specific antibodies for an extended period before undergoing apoptosis (programmed cell death).
T Lymphopoiesis
T-lymphocytes, also known as T cells, are critical players in the adaptive immune response, orchestrating various immune functions. Their development, known as T-lymphopoiesis, takes place primarily within a specialized organ called the thymus. This intricate process ensures the generation of mature T cells that are both functional (capable of recognizing foreign antigens) and tolerant (don’t attack the body’s own tissues).
The Thymus and Selection
- The thymus provides a unique microenvironment essential for T cell development and selection.
- During T-lymphopoiesis, thymocytes (T cell precursors) undergo a rigorous selection process known as positive selection and negative selection.
- Positive selection: Ensures thymocytes can interact with self-MHC molecules (major histocompatibility complex molecules) presented by thymic epithelial cells. Thymocytes unable to interact effectively are eliminated.
- Negative selection: Eliminates thymocytes that strongly bind to self-antigens presented by thymic antigen-presenting cells (APCs). This process prevents the development of autoreactive T cells that could attack the body’s own tissues.
Let’s explore the key stages of T-lymphopoiesis within the thymus
Stage | Description | Cytokines Involved | Duration (Approximate) |
Double-positive thymocyte | Early thymocyte expressing both CD4 and CD8 co-receptors on its surface. These co-receptors help T cells interact with MHC molecules on APCs. | Interleukin-7 (IL-7) | 2-4 weeks |
Positive selection | Interacts with self-MHC molecules on thymic epithelial cells. Thymocytes with weak or no interaction undergo apoptosis (programmed cell death). | IL-7 | – |
Negative selection | Interacts with self-antigens presented by thymic APCs. Thymocytes with strong binding to self-antigens undergo apoptosis. | – | – |
Single-positive thymocyte: | Selectively loses either CD4 or CD8 co-receptor, differentiating into: CD4+ T helper cell: Orchestrates various immune functions, including B cell activation and macrophage activation. CD8+ cytotoxic T cell: Directly kills infected cells and tumor cells. | IL-2 IL-15 | Variable (weeks to months) |
Additional Notes
- The duration of each stage can vary depending on individual factors and the success of selection processes.
- Once mature T cells (CD4+ or CD8+) exit the thymus and enter the bloodstream, they are considered naive T cells. These naive T cells haven’t encountered their specific antigen yet and require activation upon encountering it in the periphery (lymph nodes or other tissues).
Natural Killer Cell Development
Natural killer (NK) cells are crucial components of the innate immune system, providing a rapid first line of defense against infected cells and tumors. They develop and mature in the bone marrow, undergoing a series of stages characterized by distinct surface marker expression and functional capabilities. Although NK cells mature in the bone marrow, they don’t require migration to other organs for complete development. They reach functional maturity within the bone marrow itself.
While originating in the bone marrow like lymphocytes, NK cells diverge from the lymphoid lineage at a much earlier stage. They share a common progenitor with other lymphoid cells (CLP) but commit to the NK cell lineage through a distinct pre-NKP/ILC progenitor stage.
NK cells provide a broader immune defense and can recognize and eliminate abnormal cells like infected or tumor cells without prior sensitization. They belong to the innate immune system.
Stage | Description and Key Surface Markers | Cytokines Involved | Approximate Duration |
Common Lymphoid Progenitor (CLP) | Precursor cell with potential to differentiate into various lymphoid lineages | IL-7 | Short-lived (few days) |
Pre-NKP/ILC Progenitor (Pre-NKP/ILCP) | Commits to the NK cell lineage, expresses IL-7 receptor (IL-7R) | IL-7 | Short-lived (few days) |
Natural Killer Progenitor (NKP) | First committed NK cell precursor, expresses CD122 and NKG2D | IL-15, Flt3-ligand | 3-5 days |
Immature NK Cell (Stage A) | Early stage of NK cell development, expresses NKG2A/C and NCR1 | IL-15 | 3-5 days |
Immature NK Cell (Stage B) | Expresses NK1.1 and continues to acquire functional capabilities | IL-15 | 3-5 days |
Immature NK Cell (Stage C) | Acquires additional activating receptors (e.g., NKp46) and downregulates CD27 | IL-15 | 3-5 days |
Mature NK Cell (Stage D) | Gains complete repertoire of activating and inhibitory receptors, expresses diverse Ly49 isoforms | IL-15, IL-12, IL-18 | 7-10 days |
Terminally Mature NK Cell (Stage E) | Downregulates CD11b and expresses CD16 | IL-12, IL-15, Type I interferons | Variable |
Memory-like NK Cell (Stage F) | Subset of mature NK cells with enhanced functionality and potential for recall responses | IL-12, IL-15, Type I interferons | Variable |
Regulation of Leukopoiesis
The regulation of white blood cells (WBCs) involves a complex interplay between various feedback mechanisms, ensuring a balanced production and maintenance of these crucial components of the immune system.
Negative Feedback
This is the primary mechanism for regulating white blood cell (WBC) production. When the body encounters an infection or inflammation, it triggers the production of specific cytokines like interleukin-1 (IL-1) and interleukin-6 (IL-6). These cytokines stimulate the production of various growth factors like granulocyte-macrophage colony-stimulating factor (GM-CSF) and granulocyte colony-stimulating factor (G-CSF), leading to increased production of specific WBCs (e.g., neutrophils in bacterial infections). However, as the infection resolves or the inflammatory response subsides, the increased levels of mature WBCs themselves act as negative feedback signals.
As the number of mature WBCs of a specific type increases in the bloodstream, they release suppressor cytokines like interferon-gamma (IFN-γ), which suppress the production of growth factors by bone marrow cells. These molecules bind to receptors on hematopoietic stem cells (HSCs) and their progenitor cells in the bone marrow, inhibiting their proliferation and differentiation, thereby reducing the production of new WBCs of that specific type.
This feedback loop ensures that the body doesn’t produce an excessive amount of any particular type of WBC, preventing unnecessary strain on the immune system and potential tissue damage.
Positive Feedback
While less prevalent than negative feedback, positive feedback mechanisms can also play a role in specific situations. This typically occurs during acute infections or immune challenges to temporarily amplify the immune response.
- Inflammatory mediators: When the body encounters pathogens or tissue damage, various cells release inflammatory mediators, including cytokines like interleukin-1 (IL-1) and interleukin-6 (IL-6).
- Increased white blood cell (WBC) production: These inflammatory mediators stimulate increased production of specific WBCs, particularly neutrophils, which are crucial for fighting bacterial infections. Memory B cells and T cells generated after encountering a specific antigen can be rapidly activated upon re-exposure to the same antigen. This amplified response often leads to faster elimination of the pathogen, effectively reducing the need for further extensive WBC production.
Once the infection is controlled, the negative feedback loop takes over and regulates the production of these WBCs back to normal levels.
The Role of the Spleen
The spleen plays a crucial role in monitoring circulating blood cells, including WBCs. It acts as a reservoir for mature lymphocytes, storing them until they are needed in the immune response. During an immune response, the spleen can contract and release these stored WBCs into the bloodstream, increasing the circulating levels and aiding in immune function. Conversely, once the threat is neutralized, the spleen can relax and recapture excess circulating WBCs, contributing to the return of WBC levels to normal.
The spleen can also remove damaged or old WBCs from circulation, contributing to the overall regulation of WBC populations.
Hormonal Regulation
- Hormones such as glucocorticoids (cortisol) and sex hormones (estrogen, testosterone) can also influence the production and activity of certain WBCs to some extent. For example: Glucocorticoids (cortisol) can suppress the production of some WBCs, particularly granulocytes and monocytes, by impacting their progenitor cells in the bone marrow.
Individual Variability
It’s important to note that individual variability exists in terms of WBC counts. Factors like age, sex, ethnicity, and even daily activities can influence these levels to a certain degree. However, the feedback mechanisms mentioned above ensure that WBC counts remain within a healthy range to maintain proper immune function.
What are the types of white blood cells (WBCs)?
White blood cells (WBCs) can be broadly categorized into two main groups based on the presence or absence of cytoplasmic granules.
1. Granulocytes: These white blood cells (WBCs) have prominent, stained granules in their cytoplasm containing enzymes and other molecules critical for their function. They are further classified based on the staining properties of these granules.
- Neutrophils: The most abundant WBCs, acting as phagocytes, engulfing and destroying bacteria and fungi.
- Eosinophils: Respond to allergic reactions and parasitic infections by releasing enzymes that damage parasites.
- Basophils: Least common granulocyte, their role is less clear, but they are thought to be involved in allergic reactions, wound healing, and defense against parasites.
2. Agranulocytes: These white blood cells (WBCs) lack visible granules in their cytoplasm. They encompass a wider range of functions within the immune response.
- Lymphocytes: Key players in the adaptive immune response.
- B cells: Produce antibodies, highly specific proteins that neutralize pathogens.
- T cells: Orchestrate the immune response by activating other immune cells and directly killing infected or tumor cells.
- Monocytes: Differentiate into macrophages, powerful phagocytes that engulf debris, dead cells, and larger pathogens. They also present antigens to T cells, initiating the adaptive immune response.
- Natural Killer (NK) cells: Provide non-specific defense against virally infected cells and some tumors by directly lysing (breaking down) these target cells.
Granulocytes
Neutrophils
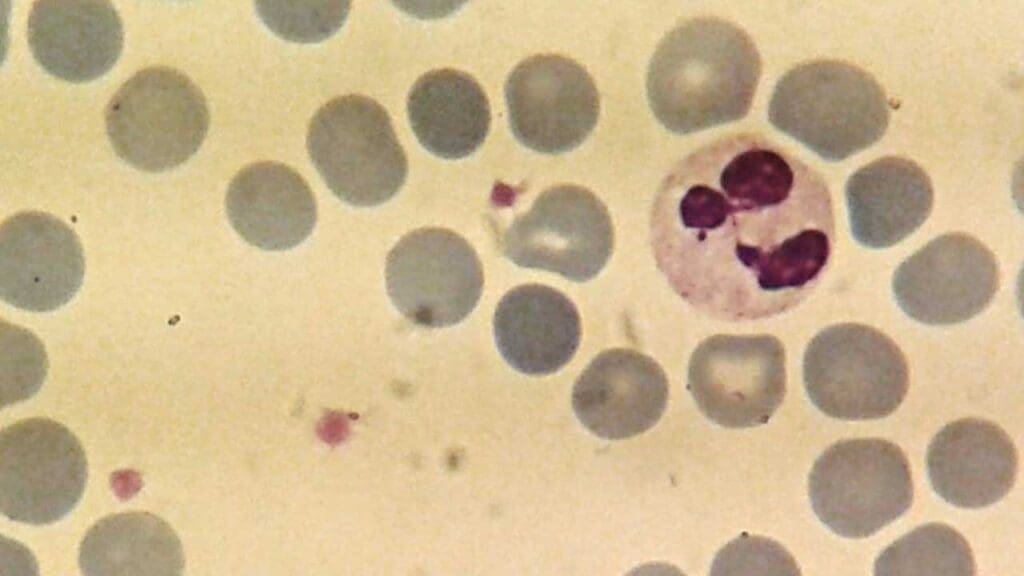
- Neutrophils are the most abundant type of white blood cell, accounting for approximately 60-70% of total white blood cells.
- They have a short lifespan, typically only lasting a few hours to a few days, but their production can be rapidly increased in response to infection.
- Their aggressive nature and powerful antimicrobial arsenal make them crucial for fighting bacterial and fungal infections. However, their excessive activation can also contribute to tissue damage in certain inflammatory conditions.
Role and function
- Phagocytosis of bacteria and fungi: Neutrophils are the first responders to infection sites and play a crucial role in phagocytosing (engulfing and destroying) bacteria and fungi. They achieve this through:
- Chemotaxis: They migrate towards chemicals released by bacteria and damaged tissues.
- Adhesion and recognition: They utilize surface molecules to adhere to and recognize pathogens.
- Phagocytosis: They engulf the pathogen using their cell membrane.
- Degranulation: They release enzymes and antimicrobial substances from their granules to kill the ingested pathogen.
- Neutrophil extracellular traps (NETs): In some situations, neutrophils can form structures called NETs, which are composed of DNA fibers and antimicrobial proteins. NETs can trap and kill pathogens, but they can also contribute to tissue damage in certain inflammatory diseases.
Structure
- Nucleus: Multi-lobed (usually 2-5 lobes), giving them a segmented appearance, which is characteristic of neutrophils.
- Cytoplasm: Abundant, pale pink, with fine granules that are not visible under light microscopy.
- Granules: These contain various enzymes and antimicrobial substances, including:
- Myeloperoxidase: Generates toxic molecules that kill pathogens.
- Lysozyme: Breaks down bacterial cell walls.
- Defensins: Antibacterial peptides.
- Lactoferrin: Binds iron, inhibiting bacterial growth.
Eosinophils
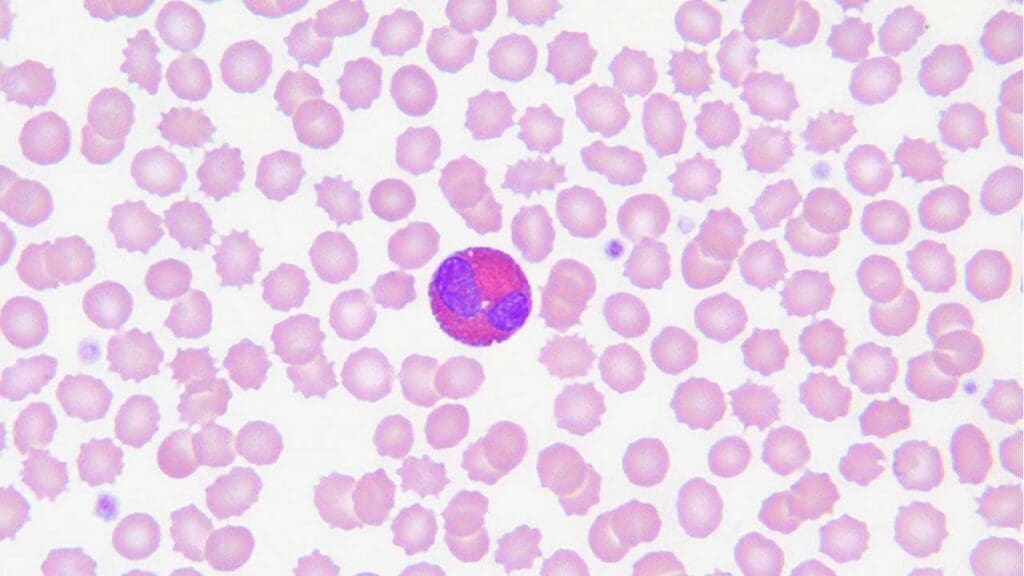
- Eosinophils are less common than neutrophils, typically accounting for 2-4% of total white blood cells.
- They have a longer lifespan compared to neutrophils, lasting for several days.
- They are recognized as important players in parasite defense and allergic reactions.
- An abnormally high number of eosinophils in the blood (eosinophilia) can be a sign of various conditions, including parasitic infections, allergic diseases, and certain autoimmune disorders.
Role and function
Eosinophils are involved in various immune responses, including:
- Parasite defense: They play a crucial role in combating parasitic infections. Their granules contain proteins and enzymes that are toxic to parasites, and they can also directly attack parasites by releasing these substances.
- Allergic reactions: Eosinophils are involved in the development of allergic reactions. They are recruited to sites of allergy and can contribute to inflammation by releasing histamine and other inflammatory mediators from their granules.
- Wound healing and tissue repair: Eosinophils participate in tissue repair processes after injury or infection. They can regulate inflammation and promote tissue regeneration.
Structure
- Nucleus: Bilobed, giving them a characteristic “spectacle-shaped” appearance.
- Cytoplasm: Pinkish, with large, coarse, orange-red granules easily visible under light microscopy.
- Granules: These contain various proteins and enzymes with diverse functions, including:
- Major basic protein (MBP): Toxic to parasites, disrupts their cell membranes.
- Eosinophil peroxidase: Generates reactive oxygen species that damage parasites.
- Eosinophil cationic protein (ECP): Toxic to parasites and some bacterial cells.
- Ribonucleases: Degrade RNA, inhibiting parasite growth and protein synthesis.
Basophils
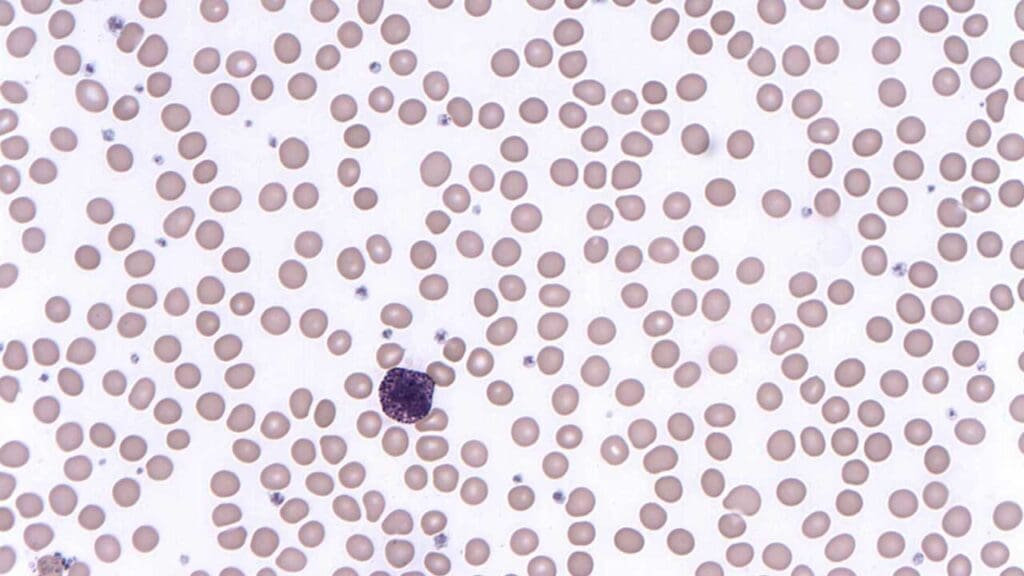
- Basophils are the least common type of granulocyte, making up less than 1% of total white blood cells.
- They have a short lifespan, typically lasting only a few hours to a few days.
- Their contribution to allergic reactions and immunomodulation is well established.
- An abnormally high number of basophils in the blood (basophilia) can be a sign of various conditions, including allergic disorders, certain infections, and some blood cancers.
Role and function
Basophils play a key role in the following processes:
- Allergic reactions: They are the only white blood cells containing histamine, a crucial mediator of allergic responses. Upon activation, basophils release histamine, leading to:
- Increased blood vessel permeability: This causes fluid leakage and tissue swelling, contributing to allergy symptoms like runny nose, itchy eyes, and wheezing.
- Smooth muscle contraction: This can cause bronchoconstriction in the lungs, leading to difficulty breathing in severe allergic reactions.
- Inflammation: Histamine attracts other immune cells and promotes inflammatory responses.
- Immunomodulation: Basophils can modulate the immune response by releasing other mediators like interleukin-4 (IL-4), which influences the activation and differentiation of other immune cells.
Structure
- Nucleus: Bilobed or S-shaped, often appearing lobed or segmented.
- Cytoplasm: Basophilic (dark blue) due to the presence of numerous basophilic granules.
- Granules: These are large and easily visible under light microscopy and contain various mediators, including:
- Histamine: Induces allergic symptoms like vasodilation, smooth muscle contraction, and increased vascular permeability.
- Heparin: An anticoagulant that prevents blood clotting.
- Proteoglycans: Contribute to inflammation and allergic reactions.
- Chondroitin sulfate: Plays a role in cell adhesion and migration.
Agranulocytes
Lymphocytes
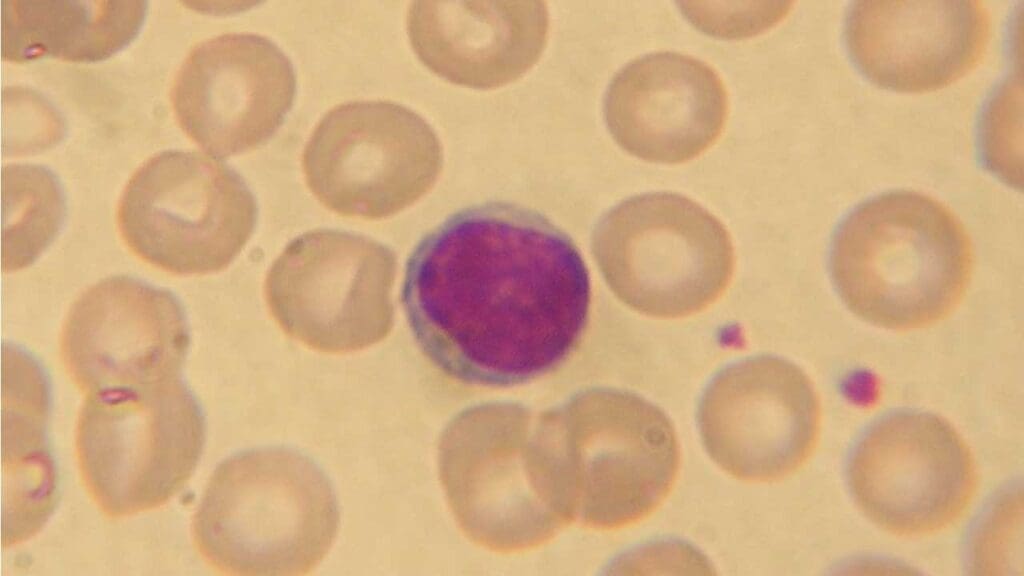
Lymphocytes are the main cells involved in the adaptive immune response, which is the body’s specific defense against particular pathogens. They are the second most abundant type of white blood cells (WBCs) They can recognize and target specific antigens (foreign molecules) from pathogens.
T cells
- T cells, also known as thymocytes due to their maturation in the thymus gland, are further classified into several subtypes with distinct functions.
- T cells are crucial for fighting intracellular pathogens like viruses and some bacteria that reside within host cells.
- They also play a role in immune surveillance, recognizing and eliminating abnormal cells, including cancer cells.
- Defects in T cell function can lead to various immunodeficiency disorders and increased susceptibility to infections and certain types of cancer.
Subtypes
- Helper T cells (Th cells): These are further categorized based on the cytokines they produce:
- Th1 cells: Drive cell-mediated immunity by activating macrophages, cytotoxic T cells, and natural killer (NK) cells. They secrete cytokines like interferon-gamma (IFN-γ) and tumor necrosis factor-alpha (TNF-α).
- Th2 cells: Promote humoral immunity by activating B cells to produce antibodies. They secrete cytokines like interleukin-4 (IL-4), IL-5, and IL-10.
- Th17 cells: Involved in inflammatory responses and defense against certain pathogens. They secrete IL-17, IL-21, and IL-22.
- T regulatory cells (Tregs): Suppress the immune response to prevent autoimmunity and maintain immune tolerance. They express the transcription factor Foxp3 and secrete IL-10 and TGF-β.
- Cytotoxic T cells (Tc cells): Also called killer T cells, they directly eliminate infected cells and cancer cells through various mechanisms, including:
- Granule exocytosis: Release perforin and granzymes to induce apoptosis (programmed cell death) in target cells.
- Fas-Fas ligand interaction: Triggering death signals through cell surface molecules.
- Memory T cells: These cells “remember” past encounters with specific antigens and can rapidly mount a stronger immune response upon re-exposure. They are further categorized into:
- Central memory T cells (TCM cells): Reside in lymphoid organs and provide long-term memory.
- Effector memory T cells (TEM cells): Circulate in the blood and tissues, offering faster but less persistent immune response.
Role and function
T cells play a central role in cell-mediated immunity, which involves the activation of various immune cells to directly target and eliminate infected or abnormal cells. They function through several key processes:
- Antigen recognition: T cells express unique antigen receptors on their surface that can bind to specific antigen fragments presented by antigen-presenting cells (APCs) such as macrophages and dendritic cells.
- T cell activation: Upon binding to the antigen-MHC complex on APCs, T cells receive co-stimulatory signals and become activated.
- Effector functions: Activated T cells differentiate into various subtypes with specific functions, such as:
- Helper T cells secrete cytokines, activating other immune cells and orchestrating the immune response.
- Cytotoxic T cells directly kill infected or cancerous cells.
- Memory T cells provide long-term immune memory and rapid response upon re-exposure to the antigen.
Structure
- Nucleus: Large, round, and dark-staining, containing the genetic material of the T cell.
- Cytoplasm: Scanty, light pink, containing various organelles for cellular functions, but lacking visible granules.
- Surface molecules: These play a crucial role in T cell function:
- T cell receptor (TCR): Unique to each T cell, it recognizes specific antigen fragments presented by MHC molecules.
- CD molecules (cluster of differentiation): These surface markers help identify different T cell subtypes and their functions.
- Co-stimulatory molecules: Essential for T cell activation, receiving signals from APCs.
B cells
- B cells, also called B lymphocytes, mature in the bone marrow and are further classified into two main subtypes; plasma cells and memory B cells.
- Antibodies can neutralize pathogens in various ways, including:
- Blocking viral attachment to host cells.
- Agglutinating (clumping) pathogens, making them easier for phagocytes to engulf.
- Activating the complement system, a cascade of proteins that can directly lyse (break open) pathogens.
- B cells also play a role in antigen presentation to T cells and can contribute to immune regulation through various mechanisms.
- Defects in B cell function can lead to immunodeficiency disorders characterized by increased susceptibility to infections and difficulty fighting off pathogens effectively.
Subtypes
- Plasma cells: These are the effector cells of the B cell lineage that are specialized for antibody production. They are short-lived and arise from activated B cells.
- Memory B cells: These cells are long-lived and “remember” specific antigens encountered during a previous infection. They can rapidly differentiate into plasma cells upon re-exposure to the same antigen, providing a faster and stronger antibody response.
Role and function
B cells are the cornerstone of humoral immunity, which involves the production of antibodies that can bind to specific antigens on pathogens, neutralizing their infectivity and facilitating their clearance from the body. They function through the following steps:
- Antigen recognition: B cells express unique antibody receptors on their surface. These receptors are specific and can bind to only one type of antigen.
- B cell activation: Upon binding to the antigen, B cells are activated by T cells or other immune factors.
- Differentiation and proliferation: Activated B cells undergo clonal expansion, dividing rapidly to produce a large number of identical daughter cells.
- Plasma cell differentiation: Most of the daughter cells become plasma cells, dedicated to antibody production.
- Antibody secretion: Plasma cells continuously produce and secrete large quantities of antibodies specific to the encountered antigen.
- Memory B cell development: A small proportion of activated B cells become memory B cells, providing long-lasting immunity.
Structure
- Nucleus: Large, round, and dark-staining, containing the genetic information of the B cell.
- Cytoplasm: Scanty, light pink, containing various organelles necessary for cellular functions, but lacking visible granules.
- Surface molecules: These play a crucial role in B cell function:
- Antibody receptors (immunoglobulins): These are Y-shaped molecules with antigen-binding sites that are unique to each B cell and recognize specific antigens.
- MHC molecules: Present antigen fragments to T cells, facilitating communication and collaboration between B and T cells.
- Co-stimulatory molecules: Receive signals from T cells or other immune cells, crucial for B cell activation.
Natural killer (NK) cells
- Unlike T cells and B cells, NK cells do not require prior exposure to an antigen to recognize and attack target cells. Their activation is primarily based on the balance between activating and inhibitory signals received from surface molecules.
- NK cells are essential for early immune defense, particularly before the adaptive immune response has fully developed or in situations where the adaptive response is compromised.
- Research is ongoing to better understand the diverse functions and potential therapeutic applications of NK cells in immunomodulation and cancer immunotherapy.
Subtypes
Natural killer (NK) cells do not have distinct subtypes. They are a single population of lymphocytes with diverse functions. However, they can be further classified based on the expression of certain cell surface markers that may correlate with their functional activities.
Role and function
NK cells are crucial components of the innate immune system, providing rapid and non-specific defense against various threats, including:
- Virus-infected cells: NK cells recognize and eliminate cells infected with viruses by detecting changes in their surface molecules or by directly interacting with viral proteins.
- Tumor cells: NK cells play a role in immunosurveillance by identifying and eliminating cancer cells due to alterations in their surface molecules or metabolic activities.
- Intracellular pathogens: NK cells can also contribute to the defense against certain intracellular bacteria and parasites through various mechanisms.
Structure
- Nucleus: Large, round, and centrally located, containing the genetic material of the NK cell.
- Cytoplasm: Abundant, pale pink to blue, containing various organelles for cellular functions. Unlike granulocytes, NK cells lack visible granules.
- Surface molecules: These play a crucial role in NK cell function:
- Activating receptors: Recognize altered self-molecules or specific ligands on infected or abnormal cells, triggering NK cell activation and cytotoxicity.
- Inhibitory receptors: Recognize healthy self-molecules, preventing NK cells from attacking healthy tissues.
- MHC class I molecules: Although not directly involved in antigen recognition, NK cells may use the presence or absence of MHC class I molecules to assess the “health” of a cell.
- Cytotoxic molecules: Upon activation, NK cells release perforin and granzymes through exocytosis, inducing apoptosis (programmed cell death) in target cells.
Monocytes
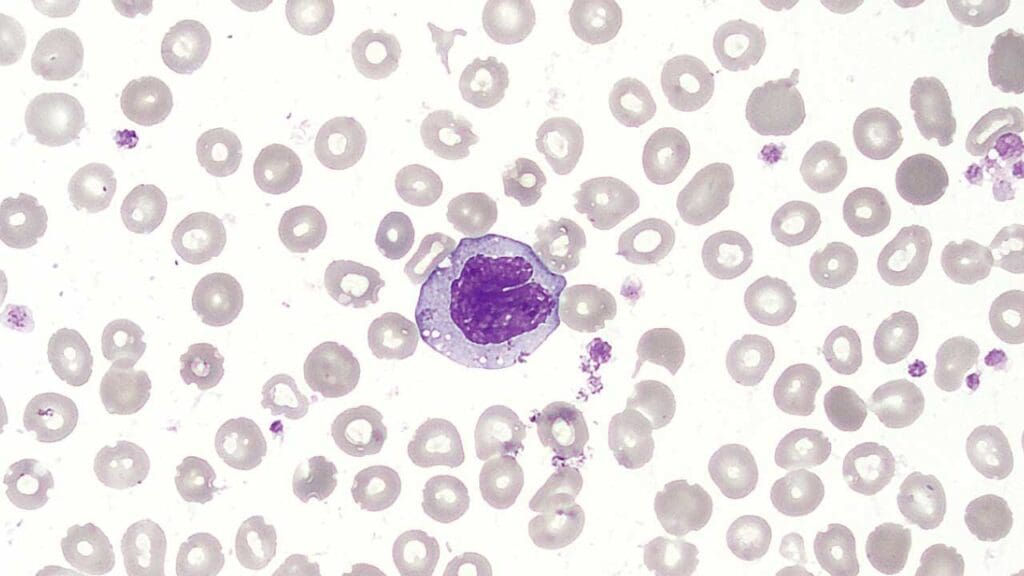
- Monocytes are the largest type of white blood cell.
- Their short lifespan in the circulation allows for rapid mobilization and differentiation into specialized cells based on tissue needs.
- They play a critical role in both innate and adaptive immunity by contributing to phagocytosis, antigen presentation, and immune regulation.
- Defects in monocyte function can lead to increased susceptibility to infections and impaired immune responses.
Subtypes
Monocytes do not have further classified subtypes. However, upon migration to tissues, they differentiate into two main cell types:
- Macrophages: These are tissue-resident phagocytes that play a crucial role in engulfing and destroying debris, pathogens, and apoptotic cells.
- Dendritic cells (DCs): These are specialized antigen-presenting cells (APCs) responsible for initiating and shaping the adaptive immune response.
Role and function
Monocytes act as precursors for these specialized cell types and play a vital role in the immune system:
- Monocyte circulation: They circulate in the bloodstream for a short period (several days) before migrating into tissues.
- Differentiation: Upon reaching their target tissues, monocytes differentiate into either macrophages or dendritic cells depending on the local signals and environment.
Structure
- Nucleus: Horseshoe-shaped or kidney-shaped, often appearing lobed or segmented.
- Cytoplasm: Abundant, pale gray, with few fine, azurophilic (staining blue) granules visible under electron microscopy. These granules contain lysosomal enzymes and other molecules involved in phagocytosis and antigen processing.
- Surface molecules: These play a role in various functions:
- Adhesion molecules: Facilitate the attachment of monocytes to endothelial cells and their migration into tissues.
- Fc receptors: Bind to the Fc portion of antibodies, allowing monocytes to recognize and phagocytize antibody-coated particles.
- Pattern recognition receptors (PRRs): Recognize specific molecules on pathogens, triggering phagocytosis and inflammatory responses.
Macrophages
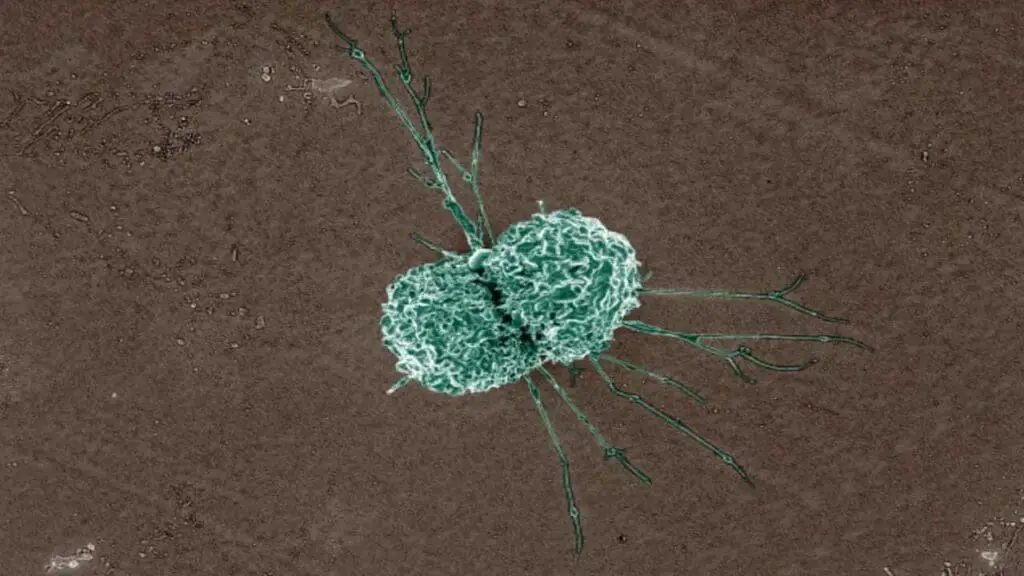
- Macrophages are highly mobile and can adapt their functions to specific tissue environments.
- They are essential for maintaining tissue homeostasis, fighting infections, and promoting wound healing.
- Dysfunctional macrophages can contribute to various inflammatory diseases, autoimmune disorders, and impaired tissue repair.
Subtypes
While monocytes do not have subtypes, macrophages exist in diverse populations throughout the body with specific functions and characteristics. These subtypes are not rigidly defined but rather reflect the adaptations and specializations that occur in different tissues.
- Alveolar macrophages: Reside in the lungs and are responsible for clearing debris and pathogens from the airways.
- Kupffer cells: Located in the liver, they phagocytize damaged red blood cells, foreign substances, and pathogens in the blood.
- Microglia: Found in the central nervous system, they play a vital role in brain development, maintenance, and immune defense.
- Osteoclasts: Responsible for bone resorption and remodeling.
- Synovial macrophages: Located in the synovial membrane of joints, they maintain joint lubrication and participate in inflammation resolution.
- Meningeal macrophages: Present in the meninges (membranes surrounding the brain and spinal cord) and contribute to immune surveillance and clearance of pathogens.
Role and function
Macrophages, derived from monocytes, are highly versatile and play numerous roles in the immune system, including:
- Phagocytosis: They engulf and destroy foreign invaders like bacteria, fungi, and cellular debris through various mechanisms, including:
- Opsonization: Binding to antibodies or complement proteins that “tag” pathogens for recognition and phagocytosis.
- Phagocytic cup formation: Extending their cell membrane to surround and engulf the target particle.
- Phagosome and lysosome fusion: Forming a phagolysosome, where ingested materials are broken down by digestive enzymes.
- Antigen presentation: They process and present antigens on their surface MHC molecules to T cells, initiating the adaptive immune response.
- Inflammation: Macrophages release various inflammatory mediators, such as cytokines and chemokines, to attract other immune cells to the site of infection or injury and initiate tissue repair processes.
- Wound healing: They contribute to wound healing by clearing debris, promoting tissue regeneration, and regulating inflammation.
- Immunoregulation: Macrophages can modulate the immune response by influencing the activation and differentiation of other immune cells.
Structure
- Nucleus: Irregular or lobulated, often appearing segmented.
- Cytoplasm: Abundant, with vacuoles and lysosomes containing ingested material.
- Surface molecules: These play a crucial role in their functions:
- Fc receptors: Bind to the Fc portion of antibodies, facilitating phagocytosis of antibody-coated particles.
- Complement receptors: Bind to complement proteins, another mechanism for recognizing and engulfing pathogens.
- Toll-like receptors (TLRs): Recognize specific molecules on pathogens, triggering inflammatory responses and antigen presentation.
- MHC molecules: Present antigen fragments to T cells.
Dendritic Cells
- DCs are strategically located in tissues exposed to the external environment, such as the skin, lungs, and gut, where they encounter various antigens.
- Their ability to capture, process, and present antigens efficiently makes them critical for generating a targeted and effective adaptive immune response.
- Research is ongoing to explore the potential of DCs in developing vaccines and immunotherapies against various diseases.
Subtypes: There are two main subtypes of dendritic cells (DCs) with distinct functions.
- Myeloid dendritic cells (mDCs)
- Develop from monocytes in the bone marrow and migrate to tissues.
- Play a crucial role in capturing and presenting antigens derived from pathogens and dead cells to T cells, thereby initiating the adaptive immune response.
- Further subclassify into various specialized populations depending on their location and function, such as Langerhans cells in the skin and interstitial DCs in various tissues.
- Plasmacytoid dendritic cells (pDCs)
- Develop in the bone marrow and lymphoid organs.
- Play a key role in antiviral immunity by producing type I interferons, which activate immune cells and inhibit viral replication.
- Can also regulate T cell responses by expressing co-stimulatory molecules and cytokines.
Role and function
DCs are the most potent antigen-presenting cells (APCs) in the immune system. They play a crucial role in linking the innate and adaptive immune responses.
- Antigen capture: DCs excel at capturing antigens from various sources, including:
- Phagocytosis: Engulfing and breaking down pathogens, dead cells, and foreign particles.
- Pinocytosis: Taking up fluids and dissolved molecules containing antigens.
- Antigen processing: DCs process captured antigens into fragments and load them onto their MHC molecules (major histocompatibility complex molecules).
- Antigen presentation: They migrate to lymphoid organs like lymph nodes, where they present antigen fragments on their MHC molecules to T cells. This interaction activates T cells and initiates the specific immune response tailored to the encountered antigen.
- Immunological tolerance: DCs can also induce tolerance to self-antigens by deleting or inhibiting self-reactive T cells, preventing autoimmune reactions.
- Regulation of immune response: DCs can influence the type of immune response (Th1, Th2, etc.) by expressing various co-stimulatory molecules and cytokines during antigen presentation.
Structure
- Nucleus: Irregular or lobulated, often appearing segmented.
- Cytoplasm: Abundant, with numerous extensions and processes (dendrites) that increase their surface area for efficient antigen capture.
- Surface molecules: These play a crucial role in DC function:
- MHC molecules: Present antigen fragments to T cells.
- Co-stimulatory molecules: Enhance T cell activation.
- Pattern recognition receptors (PRRs): Recognize specific molecules on pathogens and initiate immune responses.
- C-type lectin receptors: Bind to specific carbohydrate structures on pathogens and some self-molecules, contributing to antigen capture and processing.
Normal Ranges for White Blood Cells (WBCs)
Total WBC Count
- The normal range for a total WBC count is typically between 4,000 and 11,000 cells per microliter (μL) of blood.
- It’s important to note that these ranges can vary slightly depending on the specific laboratory performing the test, age, and other factors.
Differential Count
The differential count further breaks down the total WBC count into the percentages of each specific type of white blood cell:
- Neutrophils: 40-70%
- Lymphocytes: 20-40%
- Monocytes: 2-10%
- Eosinophils: 1-4%
- Basophils: 0.5-1%
Variations in Normal Ranges
- Age: Newborns and children typically have higher WBC counts than adults.
- Pregnancy: Pregnant women may have slightly elevated WBC counts.
- Ethnicity: African Americans tend to have slightly lower normal total WBC counts compared to other populations. This is a natural variation and not necessarily indicative of any underlying health condition. The reasons for this variation are not fully understood, but it is likely due to a combination of genetic and environmental factors.
Important Note
- A complete blood count (CBC) test, which includes the WBC count and differential, is a routine blood test used to assess overall health and screen for potential abnormalities.
White Blood Cell in Inflammation
Inflammation is the body’s natural response to injury, infection, or irritation. It’s a complex process involving various cell types, including white blood cells (WBCs), which play a crucial role in orchestrating this defense mechanism.
Different Types of White Blood Cells and their Diverse Roles
- Neutrophils: These are the first responders, rapidly migrating to the inflamed area to engulf and destroy bacteria and other pathogens through phagocytosis. They also release reactive oxygen species (ROS), potent antimicrobial molecules that can damage surrounding tissues if not controlled.
- Monocytes: They differentiate into macrophages, specialized phagocytes that engulf larger debris, dead cells, and pathogens. Macrophages also:
- Present antigens: They process and present fragments of foreign material (antigens) to T lymphocytes, activating the adaptive immune response.
- Release cytokines: These signaling molecules regulate the inflammatory response by attracting other immune cells and promoting healing.
- Lymphocytes: These specialized cells provide the adaptive immune response:
- B lymphocytes (B cells): Produce antibodies, protein molecules that bind to specific antigens, neutralizing pathogens or marking them for destruction by other immune cells.
- T lymphocytes (T cells): Orchestrate the immune response by activating other immune cells and directly killing infected or tumor cells. There are different types of T cells with specific functions, such as cytotoxic T cells (CTLs) and helper T cells (Th cells).
- Eosinophils: Primarily involved in allergic reactions and parasitic infections, they release enzymes and toxic molecules to damage parasites and contribute to allergic inflammation.
- Basophils: Their exact role remains unclear, but they are thought to be involved in allergic reactions, wound healing, and defense against parasites.
Inflammatory Mediators
- Cytokines: Signaling molecules like IL-1, IL-6, and TNF-α play a critical role in initiating and regulating the inflammatory response. They attract and activate immune cells, promote blood vessel dilation, and increase tissue permeability to facilitate the delivery of immune cells and molecules to the site of inflammation.
- Chemoattractants: These molecules, like leukotrienes, attract specific types of white blood cells to the inflamed area.
- Histamine: Released by mast cells during allergic reactions, histamine causes vasodilation, increased permeability, and smooth muscle contraction, contributing to allergy symptoms like redness, swelling, itching, and runny nose.
Side Effects and Detriments of Prolonged Inflammation
While acute, short-term inflammation is essential for healing, chronic or prolonged inflammation can be harmful.
- Fever, fatigue, and pain: These are common symptoms associated with the inflammatory response mediated by cytokines.
- Tissue damage: Uncontrolled release of ROS and inflammatory mediators can damage healthy tissues surrounding the inflamed area.
- Chronic inflammatory diseases: Unresolved or excessive inflammation can contribute to various chronic conditions like arthritis, asthma, and autoimmune diseases.
- Increased risk of cancer: Chronic inflammation can create an environment that promotes abnormal cell growth and potentially contributes to cancer development.
Additional Points
- The specific types of white blood cells involved and the extent of their involvement can vary depending on the cause of inflammation.
- Resolution of inflammation is crucial to prevent tissue damage and return to normal function. This involves the removal of inflammatory mediators and the return of affected tissues to their original state.
- Medications such as steroids can be used to suppress inflammation when it becomes excessive or harmful.
Benign Conditions and White Blood Cell (WBC) Disorders
While many conditions affecting white blood cells (WBCs) are associated with deficiencies or abnormalities in their production or function, some benign white blood cell disorders can occur.
Leukocytosis (Elevated WBC Count)
- Definition: A total WBC count exceeding 11,000 cells/μL.
- Causes:
- Physiological: Exercise, stress, pregnancy, and smoking can cause transient leukocytosis.
- Infections: Bacterial infections commonly trigger neutrophilia (increased neutrophils).
- Inflammation: Chronic inflammatory conditions like rheumatoid arthritis can lead to sustained leukocytosis.
- Medications: Certain medications like corticosteroids can cause transient leukocytosis.
- Pathophysiology: The specific mechanism of this white blood cell disorder depends on the underlying cause. For instance, during infections, cytokines released by immune cells stimulate the bone marrow to produce more WBCs, specifically neutrophils for bacterial infections.
- Clinical Presentation: Typically asymptomatic, though the underlying cause may have symptoms.
- Diagnosis: Complete blood count (CBC) test.
- Treatment: Treatment for this white blood cell disorder focuses on the underlying cause, such as antibiotics for infections or managing inflammatory conditions.
Leukopenia (Low WBC Count)
- Definition: A total WBC count below 4,000 cells/μL.
- Causes:
- Bone marrow problems: Aplastic anemia, infiltration by abnormal cells, or nutritional deficiencies can all impair WBC production.
- Increased destruction: Autoimmune diseases or splenomegaly can lead to increased WBC destruction.
- Medications: Chemotherapy or medications for autoimmune diseases can suppress bone marrow function.
- Pathophysiology: The specific mechanism of this white blood cell disorder depends on the underlying cause. For instance, aplastic anemia involves damage to the bone marrow, limiting its ability to produce all types of blood cells, including WBCs.
- Clinical Presentation: Increased susceptibility to infections, fatigue, and fever.
- Diagnosis: Complete blood count (CBC) test, bone marrow examination in some cases.
- Treatment: Treatment of this white blood cell disorder focuses on the underlying cause, such as treating underlying medical conditions or managing autoimmune diseases. In some cases, growth factors or bone marrow transplantation may be necessary.
Other Benign Causes of White Blood Cell (WBC) Disorders
Causes | Characteristics | |
Neutrophilia | • Inflammatory conditions • Infection e.g. bacterial • Leukocyte adhesion deficiency • Acute hemorrhage • Stress, Burns, Surgery • Some drugs and hormones • Hereditary neutrophilia | • Neutrophil count > 7.5 x 109/L • Left shift • Toxic granulation and vacuolation • ↑ NAP score • Dohle bodies |
Neutropenia | • Starvation, Anorexia nervosa • Chronic benign neutropenia (unknown) • Severe congenital neutropenia (elastase mutations) • Cyclic neutropenia •Drugs (e.g. myelosuppressive) • Infection, autoimmune • Nutritional deficiencies | • Neutrophil count < 2.5 x 109/L • Increased risk of infection < 0.5 x 109/L |
Eosinophilia | • Active allergic disorders (e.g. asthma and hay fever) • Dermatoses • Nonparasitic infections • Parasitic infections (e.g. hookworms) • Drugs e.g. sulphonamides • Graft vs host disease •Hypereosinophilic syndrome •Treatment with G-CSF | • Eosinophil count > 0.4 x 109/L |
Eosinopenia | • Glucocorticosteroid hormones • Acute bacterial or viral inflammation | • Eosinophil count < 0.04 x 109/L |
Basophilia | • Ulcerative colitis • Hyperlipidemia • Smallpox and chickenpox • Chronic sinusitis • Polycythemia vera | •Basophil count > 0.1 x 109/L |
Basopenia | • Hormones (e.g. corticotropin and progesterone) • During ovulation • Thyrotoxicosis | • Uncommon |
Monocytosis | • Infections (e.g. TB and bacterial endocarditis) • Fever of unknown origin • Inflammatory bowel disease • Rheumatoid arthritis • Hematological disorders (e.g. hemolytic anemia) • Collagen vascular disease e.g. RA, SLE | • Monocyte count > 1.0 x 109/L |
Monocytopenia | • Following prednisolone therapy | • Monocyte count < 0.2 x 109/L |
Lymphocytosis | • Acute infections (e.g. Rubella, pertussis, infectious mononucleosis) • Chronic infections (e.g. TB, infective hepatitis, syphilis) • Thyrotoxicosis | • Lymphocyte count > 4.0 x 109/L |
Lymphocytopenia | • Cytotoxic drugs • Irradiation • HIV infection | • Lymphocyte count < 1.5 x 109/L |
May-Hegglin anomaly | Autosomal dominant genetic mutation in the MYH9 gene on chromosome 22q12-13. This gene is involved in the production of a protein essential for proper platelet development and function. | • Thrombocytopenia: Variable decrease in the number of platelets in the blood, leading to an increased risk of bleeding. • Giant platelets: Abnormally large platelets with abnormal morphology. • Döhle-like inclusions: Large blue inclusions within neutrophils, eosinophils, basophils, and monocytes. These inclusions are thought to be remnants of abnormal granules. Usually asymptomatic, but some individuals may experience easy bruising or bleeding. |
Pelger-Huet anomaly | Autosomal dominant genetic mutation in the ELANE gene on chromosome 19p13. This gene is involved in the regulation of neutrophil nuclear shape during maturation. | • Hyposegmentation: Neutrophils with abnormally shaped nuclei, appearing less segmented than usual. • No functional impairment: Despite the abnormal appearance, the neutrophils typically function normally and do not cause any symptoms or health problems. |
Chediak-Higashi syndrome | Autosomal recessive genetic mutation in the CHS1 gene on chromosome 1q42.1-q42.2. This gene encodes a protein involved in the formation and function of lysosomes, which are cellular compartments responsible for waste disposal and recycling. | • Partial oculocutaneous albinism: Reduced pigmentation in the skin, hair, and iris of the eyes. • Photophobia: Increased sensitivity to light. • Neurological symptoms: May include tremors, nystagmus (involuntary eye movements), and developmental delay in some individuals. • Increased risk of infections: Due to impaired function of immune cells, individuals with CHS are more susceptible to recurrent bacterial infections. • Giant lysosomes: Neutrophils and other immune cells contain abnormally large lysosomes, hindering their ability to effectively fight infections. |
Severe Burns
Severe burns trigger a complex and dynamic response from the immune system, including significant changes in white blood cell (WBC) counts.
Early Stage (First few days)
- Initial Increase: Immediately after a burn, there’s often a transient increase in total WBC count, primarily driven by neutrophils. This initial rise can be attributed to:
- Stress hormones: The body releases stress hormones like cortisol, which can stimulate the bone marrow to produce more WBCs.
- Inflammatory response: The burn injury triggers an inflammatory response, leading to the release of cytokines that further stimulate neutrophil production.
Later Stage (Days to weeks)
- Leukopenia: Following the initial rise, a significant decrease in total WBC count, particularly neutrophils, can occur. This leukopenia can last for several days to weeks.
- Possible reasons for leukopenia:
- Depletion of bone marrow reserves: The initial surge in neutrophil production can exhaust the bone marrow’s readily available reserves.
- Increased apoptosis: Neutrophils at the burn site may undergo increased apoptosis (programmed cell death).
- Sequestration: Some neutrophils may become trapped in damaged tissues or the lungs, contributing to the apparent decrease in circulating neutrophils in the blood.
Long-Term
- Gradual recovery: Over time, the WBC count and composition typically gradually return towards normal levels as the body recovers from the burn and the bone marrow replenishes its reserves.
Malignant White Blood Cell (WBC) Disorders
Malignant white blood cell (WBC) disorders, also known as leukemias, involve the uncontrolled growth and abnormal development of white blood cells. This abnormal proliferation disrupts the normal production of healthy blood cells and can impair the immune system’s ability to fight infections effectively.
Acute Myeloid Leukemia (AML)
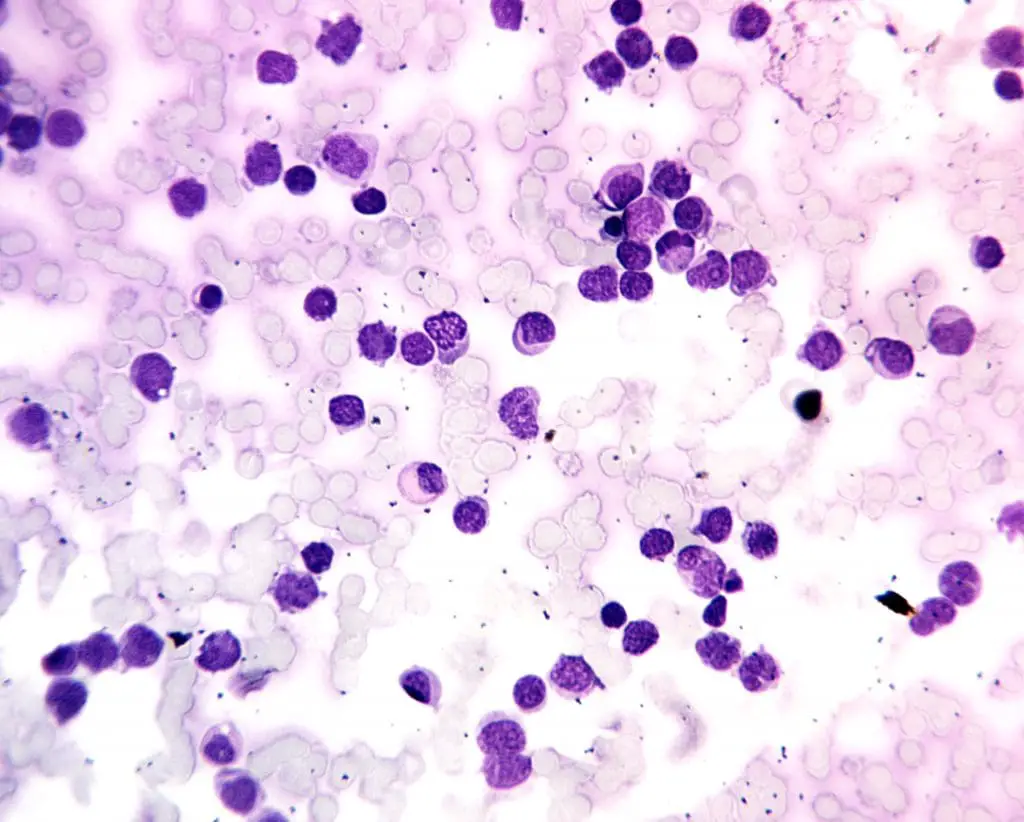
- Definition: A white blood cell disorder that has rapidly progressing cancer of the bone marrow and blood characterized by the abnormal proliferation of immature myeloid cells (precursors to various types of white blood cells (WBCs), red blood cells, and platelets).
- Causes: The exact cause of this white blood cell disorder is often unknown, but risk factors include exposure to radiation, certain chemicals (benzene), and some genetic predispositions.
- Pathophysiology: Mutations in genes that regulate cell growth and differentiation cause uncontrolled proliferation of immature myeloid cells in this white blood cell disorder. These abnormal cells crowd out healthy blood cell production and impair immune function in this white blood cell disorder.
- Clinical presentation: Fatigue, fever, easy bruising or bleeding, pale skin, weight loss, and frequent infections.
- Diagnosis: Complete blood count (CBC), bone marrow biopsy and aspiration, cytogenetic analysis, and molecular studies.
- Treatment: Intensive chemotherapy, with or without bone marrow transplantation in some cases.
Acute Lymphoblastic Leukemia (ALL)
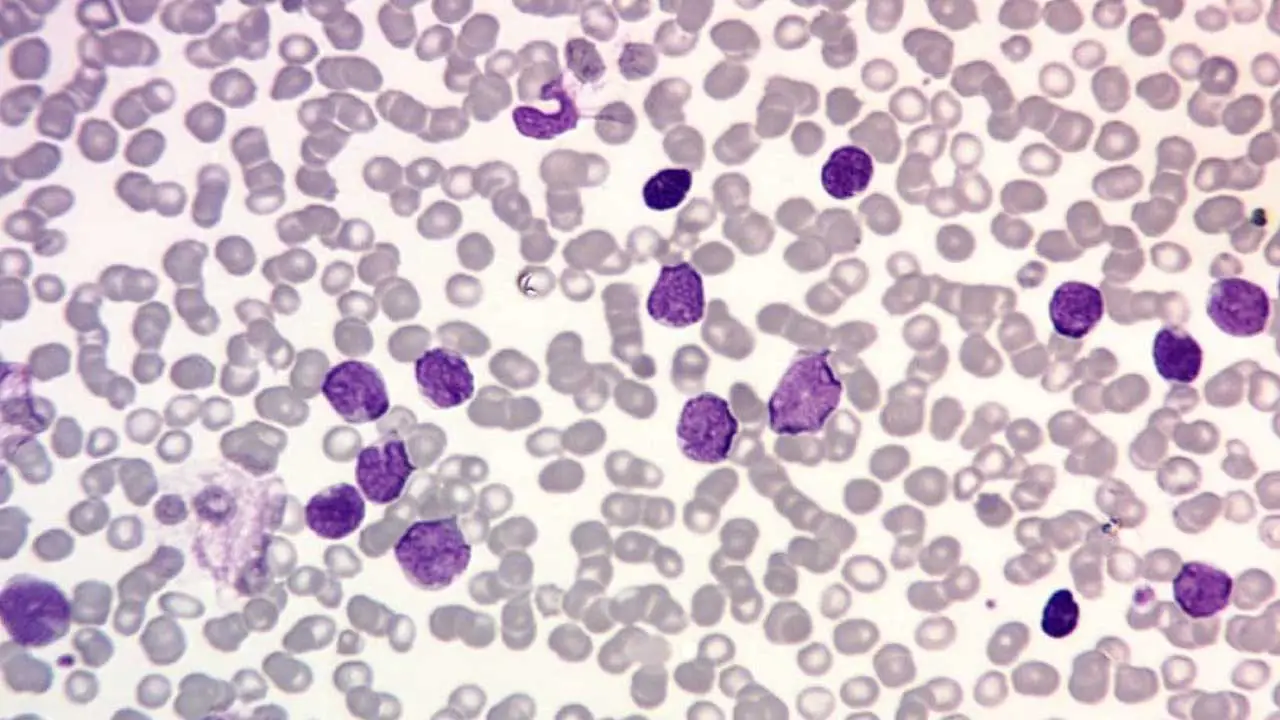
- Definition: This white blood cell disorder is a rapidly progressing cancer that originates in the bone marrow and blood, affecting lymphocytes (a type of white blood cell (WBC) responsible for the immune response).
- Causes: Similar to AML, the exact cause of this white blood cell disorder is often unknown, but risk factors include exposure to radiation, certain chemicals (benzene), and some genetic predispositions.
- Pathophysiology: Mutations in genes regulating lymphocyte development lead to uncontrolled growth of immature lymphocytes in this white blood cell disorder. These abnormal cells impair the production of healthy lymphocytes and weaken the immune system.
- Clinical presentation: Similar to AML, but may include swollen lymph nodes and bone pain.
- Diagnosis: Similar to AML, including blood tests, bone marrow examination, and other tests to determine the subtype of ALL.
- Treatment: Chemotherapy, with or without other therapies like radiation therapy or targeted therapies depending on the specific subtype of ALL.
Chronic Myeloid Leukemia (CML)
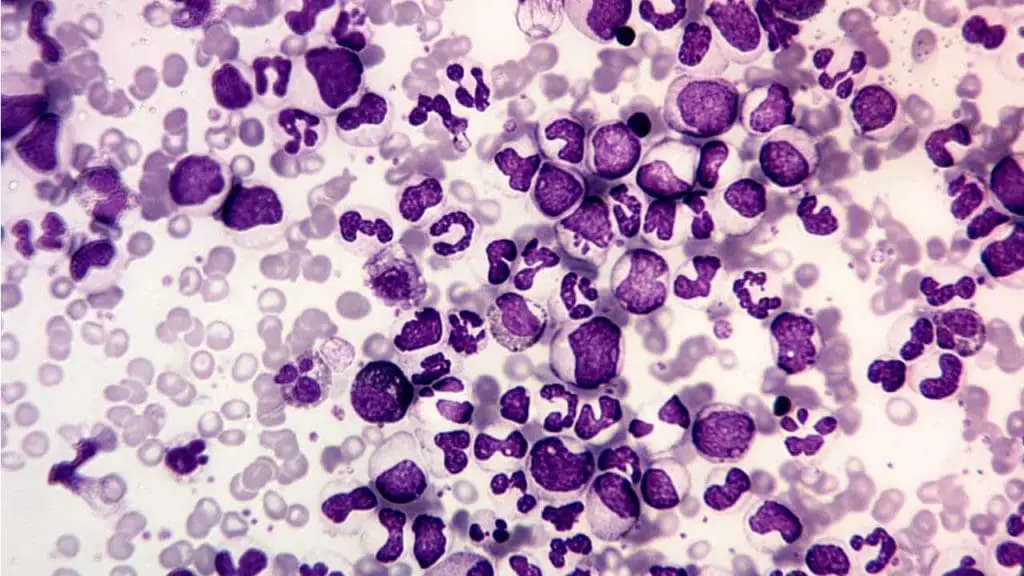
- Definition: This white blood cell disorder is a slow-growing cancer affecting the bone marrow and blood, characterized by an abnormal Philadelphia chromosome and uncontrolled growth of mature and immature myeloid cells.
- Causes: The primary cause of this white blood cell disorder is the development of the Philadelphia chromosome, a translocation of genetic material between chromosomes 9 and 22. This translocation activates an abnormal protein that promotes uncontrolled cell growth.
- Pathophysiology: The Philadelphia chromosome leads to uncontrolled proliferation of both mature and immature myeloid cells, disrupting normal blood cell production and potentially causing other complications in this white blood cell disorder.
- Clinical presentation: May have no symptoms initially, but can progress to fatigue, weight loss, and abdominal discomfort as the spleen enlarges.
- Diagnosis: Similar to AML, including blood tests, bone marrow examination, and cytogenetic analysis to identify the Philadelphia chromosome.
- Treatment: Targeted therapy with tyrosine kinase inhibitors (TKIs) is the mainstay of treatment, aiming to suppress the abnormal protein activity and control the disease.
Chronic Lymphocytic Leukemia (CLL)
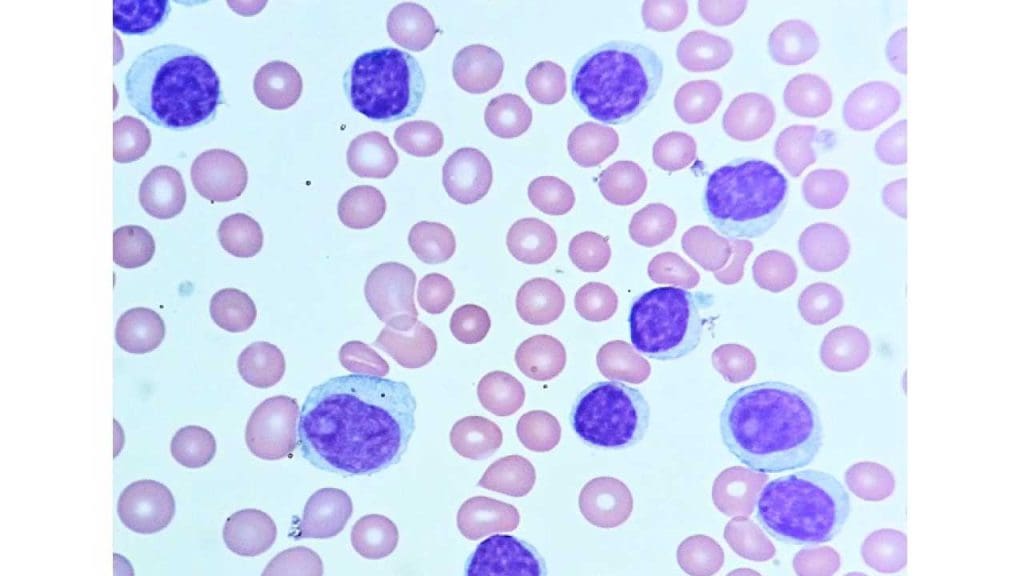
- Definition: This white blood cell disorder is a slow-growing cancer affecting the bone marrow and blood, characterized by the abnormal proliferation of mature B lymphocytes (a type of lymphocyte).
- Causes: The exact cause is unknown of this white blood cell disorder, but risk factors include age, family history, and exposure to certain chemicals.
- Pathophysiology: Mutations in genes regulating B lymphocyte development lead to uncontrolled growth of mature B lymphocytes in this white blood cell disorder. These abnormal cells accumulate in the bone marrow and blood, interfering with the production of healthy blood cells and potentially suppressing the immune response.
- Clinical presentation: May have no symptoms initially, but can progress to fatigue, enlarged lymph nodes, and weight loss.
- Diagnosis: Similar to other leukemias, including blood tests, bone marrow examination, and flow cytometry to identify the specific type of abnormal lymphocyte.
- Treatment: May not require immediate treatment for individuals with no symptoms; treatment options for symptomatic individuals can include chemotherapy, immunotherapy, or targeted therapy depending on the specific characteristics and progression of the disease.
Frequently Asked Questions (FAQs)
Is lymphoma a disorder of the white blood cell?
While lymphoma involves abnormal lymphocytes, which are a type of white blood cell (WBC), it’s not technically classified as a white blood cell disorder. Here’s the key distinction:
- Origin: Unlike leukemias, which directly originate in the bone marrow, the primary site for blood cell production, lymphomas arise in lymphoid tissues like lymph nodes, spleen, and thymus. These tissues are responsible for housing and maturing lymphocytes, but they are not the primary site for their production.
- Malignancy: Although leukemias and lymphomas are both malignant conditions affecting the immune system, they involve different aspects of the WBC system.
- Leukemias: These white blood cell disorder are cancers of the bone marrow itself, affecting the entire spectrum of WBCs at various stages of development.
- Lymphomas: These are cancers originating in lymphoid tissues, primarily affecting mature lymphocytes within these tissues.
Therefore, even though lymphomas involve the abnormal growth of lymphocytes, which are a type of white blood cell, they are considered distinct entities arising from the lymphatic system, not the bone marrow, and primarily affecting mature lymphocytes within these tissues.
Are white blood cell counts high with cancer?
A high white blood cell (WBC) count (leukocytosis) is not always indicative of cancer. WBC count can fluctuate due to various factors, and cancer is just one possibility.
Cancers with High WBC Count
- Leukemias: These are cancers of the bone marrow itself, directly affecting the production of WBCs. Most leukemias, particularly acute leukemias, typically present with a very high WBC count. This is because the bone marrow produces abnormal and immature WBCs that flood the bloodstream.
- Metastatic Cancers: In some cases, cancers that have spread (metastasized) from their original location can trigger an inflammatory response, leading to an elevated WBC count. This is not universal and depends on the specific cancer and the extent of spread.
Cancers with Normal or Low WBC Count
- Solid Tumors: Many solid tumors, such as lung cancer, breast cancer, or colon cancer, may not cause significant changes in WBC count. Their impact on the bone marrow and overall WBC production is often minimal.
- Cancers that Suppress the Immune System: Some cancers can actually suppress the immune system, leading to a low WBC count. This can make individuals more susceptible to infections. Examples include certain lymphomas and advanced stages of some cancers.
Additional Considerations
- Infection: Even in the absence of cancer, infections are a common cause of elevated WBC counts.
- Other Inflammatory Conditions: Autoimmune diseases and chronic inflammatory conditions can also lead to high WBC counts.
Overall
A WBC count is just one piece of the puzzle when evaluating a potential cancer diagnosis. Other factors like symptoms, medical history, and other diagnostic tests are crucial for a complete picture.
Can white blood cell count fluctuate?
Yes, white blood cell (WBC) counts can fluctuate naturally throughout the day and in response to various factors.
Natural Fluctuations
- Circadian Rhythm: WBC counts tend to be higher in the morning and gradually decrease throughout the day, reaching a low point at night. This fluctuation is likely related to hormonal changes and activity levels.
- Age: Newborns typically have higher WBC counts compared to adults. WBC counts generally decrease with age.
- Stress: Physical or emotional stress can cause a temporary increase in WBC count due to the release of hormones like cortisol.
- Exercise: Strenuous exercise can transiently elevate WBC count, as the body prepares to combat potential micro-injuries or inflammation.
Fluctuations Due to Medical Conditions
- Infection: This is a common cause of elevated WBC count. The body increases WBC production to fight off the invading pathogens.
- Inflammation: Chronic inflammatory conditions like rheumatoid arthritis can lead to a persistent elevation in WBC count.
- Immunosuppression: Certain medications or medical conditions can suppress the immune system, resulting in a low WBC count. This can increase susceptibility to infections.
- Allergies: During an allergic reaction, the body releases substances like histamine, which can trigger a temporary increase in WBC count, particularly eosinophils.
Other Factors
- Smoking: Can lead to a slight increase in WBC count due to chronic inflammation in the lungs.
- Pregnancy: WBC counts often increase during pregnancy as the body prepares for childbirth.
It’s important to note that
- The extent of fluctuation can vary depending on the individual and the underlying cause.
- A single WBC count outside the normal range may not necessarily be a cause for concern. However, a persistent elevation or reduction, especially when accompanied by other symptoms, should be evaluated.
Which antibiotics decrease white blood cell count?
While some antibiotics can have various side effects, it’s important to understand that not all antibiotics decrease white blood cell (WBC) count. In fact, most antibiotics are generally well-tolerated and do not cause significant changes in WBC count.
However, certain antibiotics are known to have a potential association with reducing WBC count in rare cases. These include:
- Beta-lactam antibiotics: This is a large class of antibiotics that includes penicillins, cephalosporins, and others. While uncommon, some individuals may experience a decrease in neutrophils (neutropenia) upon receiving these antibiotics.
- Chloramphenicol: This antibiotic can suppress the bone marrow, leading to a decrease in all types of blood cells, including WBCs. However, its use is generally limited due to the potential for serious side effects.
- Vancomycin: This antibiotic can rarely cause neutropenia, especially in high doses or prolonged use.
Disclaimer: This article is intended for informational purposes only and is specifically targeted towards medical students. It is not intended to be a substitute for informed professional medical advice, diagnosis, or treatment. While the information presented here is derived from credible medical sources and is believed to be accurate and up-to-date, it is not guaranteed to be complete or error-free. See additional information.
References
- Goldberg S, Hoffman J. Clinical Hematology Made Ridiculously Simple, 1st Edition: An Incredibly Easy Way to Learn for Medical, Nursing, PA Students, and General Practitioners (MedMaster Medical Books). 2021.
- Keohane EM, Otto CN, Walenga JM. Rodak’s Hematology 6th Edition (Saunders). 2019.
- Blumenreich MS. The White Blood Cell and Differential Count. In: Walker HK, Hall WD, Hurst JW, editors. Clinical Methods: The History, Physical, and Laboratory Examinations. 3rd edition. Boston: Butterworths; 1990. Chapter 153.