TL;DR
The coagulation cascade is a complex series of biochemical reactions that occur in the blood when a vessel is injured. It’s a tightly regulated process that involves numerous proteins, called coagulation factors, working together to form a blood clot and stop bleeding.
- Two Pathways ▾: The intrinsic and extrinsic pathways, triggered by internal and external damage respectively, converge in the common pathway, culminating in fibrin clot formation.
- Key players ▾: Zymogens, serine proteases, cofactors, calcium ions.
- Fibrin Formation ▾: The fibrin clot acts as a natural plug, stopping blood loss and allowing wound healing to begin.
- Regulation and Inhibition ▾: The process is tightly controlled by natural anticoagulants like antithrombin III, preventing excessive clot formation and maintaining balance.
- Related Bleeding Disorders: Hemophilia, factor V deficiency, protein C/S deficiency etc.
*Click ▾ for more information
Introduction
Hemostasis is the body’s remarkable process of stopping blood flow at a site of injury. It’s a delicate balance between maintaining smooth blood flow throughout the body and preventing excessive bleeding when a vessel is damaged. This article covers the importance of secondary hemostasis or the coagulation cascade in more detail.
The Two Phases of Hemostasis
Hemostasis operates in two distinct phases, working together seamlessly to seal the breach:
- Primary Hemostasis: This is the initial response, activated within seconds of injury. Platelets, tiny disc-shaped cells in your blood, stick to the damaged vessel wall like sticky patches, forming a temporary plug to slow the blood flow.
- Secondary Hemostasis (Coagulation Cascade): This is the reinforcement phase, taking over from platelets within a few minutes. A complex cascade of proteins called coagulation factors kicks in, creating a fibrin mesh that strengthens and stabilizes the platelet plug into a permanent clot.
The blood coagulation cascade plays a vital role in maintaining blood flow and preventing excessive bleeding. It’s like a powerful backup system, ensuring the platelet plug is adequately reinforced and stabilized to effectively seal the wound.
Hemostasis is a finely balanced process. While clotting is crucial to stop bleeding, excessive clotting can be dangerous, blocking blood flow and causing tissue damage. To prevent this, the body has a built-in system of anticoagulants, proteins that work to break down and remove clots once they are no longer needed. It’s like a constant tug-of-war, ensuring the clot forms where and when needed but doesn’t overstay its welcome.
Coagulation Factors
- Zymogens: These are inactive precursors of coagulation factors. They wait patiently in the blood until a trigger, like an injury, sets them off.
- Serine proteases: These are enzymes that snip specific protein bonds in other factors, activating them.
- Cofactors: These are proteins that bind to and stabilize serine proteases, ensuring they work efficiently.
- Calcium ions: These are the “spark plugs,” essential minerals that bind to specific sites on coagulation factors, enabling them to function properly.
Numbering system and nomenclature used for coagulation factors
Coagulation factors might seem like a jumble of names, but there’s actually a logic behind it! They’re numbered based on their order of discovery, with Roman numerals for historical reasons (e.g., factor VII was discovered before factor VIII). Sometimes, lowercase letters are added to indicate different activation states (e.g., factor Xa is the activated form of factor X).
Main coagulation factors, their activation mechanisms, and functions.
Factor | Activation Mechanism | Function |
Tissue Factor (TF) | Injury exposure | Initiates extrinsic pathway |
Factor VII (FVII) | TF binding, calcium | Activates factor X |
Factor X (FX) | FVIIa cleavage, calcium | Activates factor IX and prothrombin |
Factor IX (FIX) | FXa cleavage, calcium, factor VIIIa | Activates factor X |
Factor VIII (FVIII) | Factor IXa cleavage, calcium | Cofactor for factor IXa |
Prothrombin (FII) | FXa cleavage, calcium | Converted to thrombin |
Thrombin (FIIa) | Cleaves fibrinogen | Forms fibrin clot, activates factor XIII |
Fibrinogen (Fg) | Thrombin cleavage | Forms fibrin mesh, strengthens clot |
Factor XIII (FXIII) | Thrombin activation, calcium | Stabilizes fibrin clot by cross-linking |
Protein C (PC) | Thrombin activation | Inhibits factors Va and VIIIa, prevents excessive clotting |
Coagulation Cascade
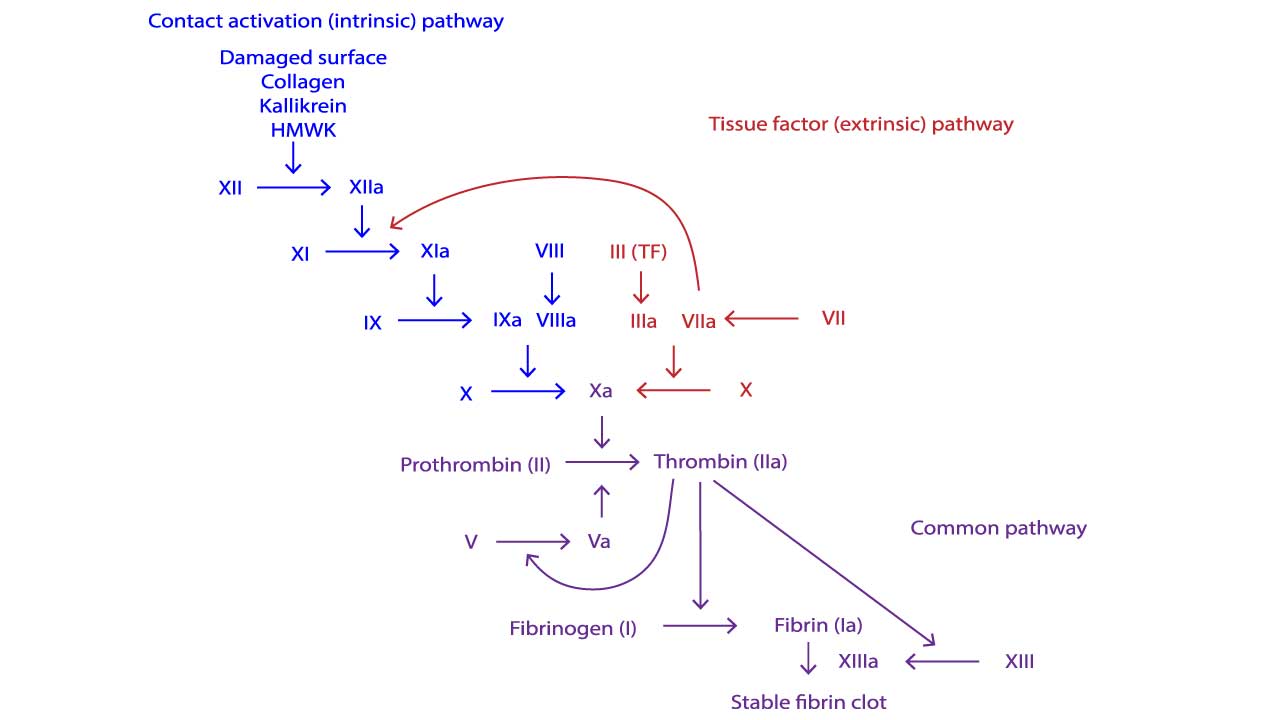
Intrinsic Pathway
The intrinsic pathway, activated by internal factors or contact with collagen following injury, involves a cascade of reactions. Once triggered, factor XII activates factor XI, which then activates factor IX. Factor IX, along with factor VIII and calcium, forms a complex that activates factor X. This activated factor X, alongside factor Va and calcium, then converts prothrombin (factor II) into thrombin, the enzyme that drives clot formation by converting fibrinogen into fibrin, the mesh of fibers that forms the clot.
Extrinsic Pathway
The extrinsic pathway, triggered by external injury, initiates clotting faster than the intrinsic pathway. When tissue factor (factor III) exposed by damage comes in contact with circulating factor VII, they activate factor X. This activated factor X then joins forces with factors Va and calcium to convert prothrombin (factor II) into thrombin, the enzyme responsible for clot formation. This kickstarts the common pathway, leading to fibrin clot formation.
Common Pathway
In a so-called prothrombinase complex, activated factor X together with activated factor V promotes a downstream enzymatic cleavage of prothrombin, which yields small amounts of thrombin.
Thrombin is a pleiotropic, central coagulation enzyme that not only converts fibrinogen into fibrin but also has a substantial role in the activation of platelets and activates factor XIII to induce fibrin polymerization, a fundamental process for the formation of a stable clot, or thrombus. Furthermore, by supporting positive-feedback activation of the upstream factors V, VIII, and XI, thrombin plays a crucial part in the amplification and propagation phases of coagulation.
The activated platelet surface is also a critical catalyst for the coagulation cascade. Platelets actively participate in the clotting process by introducing extra amounts of tissue factor, factor V, fibrinogen, and factor XIII into the system, derived from various local sources (fibrinogen and factors V and XIII stored in α granules), and facilitating the direct activation of factor XI by thrombin and the subsequent activation of factor IX on the platelet surface. Factor IXa forms the so-called tenase complex together with factor VIIIa, thereby igniting a burst of additional thrombin generation, which is essential in forming sufficient fibrin and sealing the defect.
Cell-based Model
The traditional waterfall model of the coagulation cascade, with its distinct intrinsic and extrinsic pathways, has served us well for decades. However, recent advancements have led to the development of a more nuanced and physiologically accurate model: the cell-based model. This revised model emphasizes the crucial role of cells in orchestrating the coagulation process, offering a more comprehensive understanding of hemostasis in vivo.
The Traditional Model
- Independent Pathways: Imagine two separate waterfalls, intrinsic and extrinsic, converging to form a large clot. The intrinsic pathway starts within the vessel, while the extrinsic pathway is triggered by tissue damage.
- Plasma-Centric: Factors dance primarily in the plasma, with minimal interaction with cells. They activate each other in a domino-like fashion, ultimately leading to fibrin clot formation.
- Emphasis on Factors: Each step revolves around specific coagulation factors, numbered and neatly categorized (e.g., factor VIII, factor XIII).
Key Features of the Cell-Based Model
- Cellular Control: The initiation and amplification of coagulation are primarily localized on the surfaces of activated platelets and vascular endothelial cells, rather than occurring freely in the plasma.
- Three Overlapping Phases: The cascade is divided into three phases: initiation, amplification, and propagation, instead of the traditional two pathways. Each phase involves specific cell types and interactions.
- Tissue Factor (TF): This key initiator is not just present in injured tissues but also expressed by activated endothelial cells and circulating monocytes, highlighting its broader role in triggering coagulation.
- Fibrinogen Binding: Platelets play a crucial role in confining the fibrin clot formation to the site of injury by binding fibrinogen to their surfaces.
Differences from the Traditional Model
- No Distinct Pathways: The cell-based model emphasizes the interconnectedness and overlap between the traditional intrinsic and extrinsic pathways. Both pathways contribute to the same phases, occurring on the surfaces of activated cells.
- Emphasis on Platelets: Platelets are not just passive participants but actively contribute to the initiation, amplification, and propagation of the cascade through their surface-based reactions.
- Localized Control: Coagulation is tightly localized to the site of injury, minimizing the risk of inappropriate clot formation elsewhere in the vasculature.
Benefits of the Cell-Based Model
- Improved Understanding of Hemostasis: The cell-based model provides a more realistic representation of how coagulation occurs in vivo, leading to a better understanding of physiological hemostasis.
- Diagnosis and Treatment of Bleeding Disorders: This model can offer new insights into the pathogenesis of bleeding disorders and aid in the development of targeted therapies.
- Personalized Medicine: By understanding the cellular and molecular mechanisms involved in blood coagulation, we can pave the way for personalized medicine approaches to bleeding and thrombotic disorders.
The cell-based model doesn’t completely discard the traditional waterfall framework of the coagulation cascade. They represent different viewpoints: waterfall emphasizes the sequence of reactions, while cell-based focuses on the actors and the stage.
Fibrin Formation and Clot Stabilization
The climax of hemostasis lies in the transformation of a soluble protein called fibrinogen into a robust fibrin mesh that seals the wound. This intricate process involves two crucial players: activated thrombin and activated factor XIII, all meticulously orchestrated by calcium ions.
From Fibrinogen to Fibrin Monomers
Thrombin cleaves fibrinogen, a large protein in the plasma, into smaller units called fibrin monomers. This cleavage isn’t haphazard. Thrombin targets specific peptide bonds within fibrinogen, releasing fibrinopeptides A and B. These peptides act like anchors, keeping the monomers soluble and preventing premature polymerization.
Thrombin also exposes hidden binding sites on the fibrin monomers. These sites, like molecular velcro, allow the monomers to connect with each other, setting the stage for the next critical step.
Building a Stable Mesh
Activated factor XIII, another vital player, binds to exposed lysine residues on the fibrin monomers, acting like a molecular sewing kit. Factor XIII then catalyzes the formation of covalent bonds between these lysines, stitching the fibrin monomers together into a stable, insoluble mesh. This fibrin mesh is the core of the clot, the physical barrier that plugs the leak and prevents further blood loss.
Calcium Ions
Calcium ions, though not directly involved in the cleavages or cross-linking, play a crucial supporting role. They bind to specific sites on both thrombin and factor XIII, stabilizing their structures and facilitating their interactions with fibrinogen and fibrin monomers.
The conversion of fibrinogen to fibrin and its subsequent cross-linking are crucial for several reasons:
- Clot strength and stability: The fibrin mesh provides a strong, insoluble barrier against blood flow, ensuring the clot remains intact until the wound heals.
- Localized clot formation: The process is tightly regulated by thrombin and factor XIII, preventing unwanted clot formation in the vasculature.
- Wound healing: The fibrin mesh creates a scaffold for tissue repair, promoting cell migration and blood vessel formation at the wound site.
Coagulation Inhibition
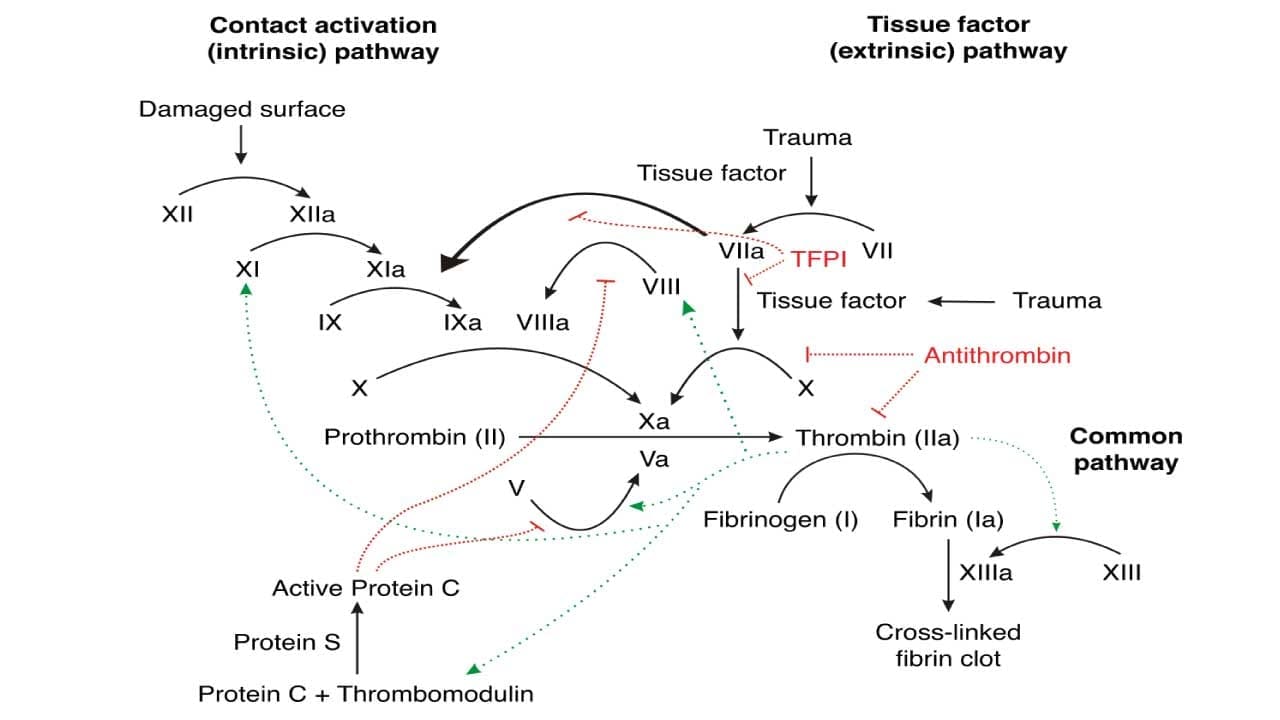
It is just as important that coagulation be limited to the site of injury, because its continued extension to adjacent normal vessels could occlude blood flow which may be fatal. Thus there are several molecules and pathways which limit the extension of clot formation.
- Tissue factor pathway inhibitor (TFPI) is synthesized in endothelial cells and is present in plasma and platelets. It blocks the extrinsic pathway after its initial activation by binding first to factor Xa. This binary factor Xa/TFPI complex then docks onto and inactivates factor VIIa/tissue factor complexes, shutting down the extrinsic pathway after a small amount of factor Xa and subsequently thrombin, have been generated. This is sufficient to activate factor VIII and factor C, enabling the intrinsic pathway to provide a burst of procoagulant activity.
- Antithrombin is the most important plasma protease inhibitor in regulating hemostasis. Upon binding to endogenous glycoconjugates on the surface of endothelial cells or the anticoagulant heparin, antithrombin undergoes a change in conformation that vastly increases its activity as a serine protease inhibitor. It inactivates not only thrombin but also factors VIIa, IXa, Xa and XIa as they are carried in the blood away from the developing thrombus.
- Protein C, in the presence of its cofactor, protein S, is activated by thrombin bound to thrombomodulin on the surface of normal endothelial cells. Protein C and protein S both undergo vitamin K-dependent carboxylation and share other structural features with factors VII, IX, X and prothrombin. Activated protein C reduces the coagulation cascade by specifically inactivating factors Va and VIIIa.
- Thrombomodulin is present on the surface of vascular cells. Thrombomodulin binds to thrombin with high affinity, forming a complex that effectively neutralizes its clotting activity. Thrombomodulin-thrombin complex activates protein C, a potent anti-coagulation agent. Protein C then inactivates factors V and VIII, key players in the coagulation cascade, further dampening clot formation. Beyond stopping clot formation, thrombomodulin plays a role in breaking down existing clots. It activates thrombin-activatable fibrinolysis inhibitor (TAFI), an enzyme that enhances the activity of plasmin, the main player in fibrinolysis.
Disorders and Treatments
The finely tuned blood coagulation cascade ensures our blood flows smoothly and wounds heal efficiently. But what happens when some players are missing or malfunctioning? This is where bleeding disorders come in, a collection of conditions characterized by excessive or uncontrolled bleeding.
Intrinsic Pathway
- Hemophilia A (Factor VIII deficiency): Most common type of hemophilia, causing prolonged bleeding after injuries.
- Hemophilia B (Factor IX deficiency): Similar to hemophilia A, but less frequent.
- Hemophilia C (Factor XI deficiency): Less common than A or B, with variable bleeding severity.
- Factor XII deficiency: Rare, often asymptomatic, but can cause prolonged bleeding after surgery.
- Prekallikrein deficiency: Very rare, associated with mild bleeding tendency.
- High Molecular Weight Kininogen (HMWK) deficiency: Extremely rare, with variable bleeding symptoms.
Common Pathway
- Prothrombin deficiency (Factor II deficiency): Rare, causing severe bleeding, often requiring lifelong vitamin K therapy.
- Factor V deficiency: Can be inherited or acquired, causing mild to severe bleeding depending on the severity of the deficiency.
- Factor VII deficiency: Rare, causing severe bleeding, often requiring factor VII concentrate replacement.
- Factor X deficiency: Rare, with variable bleeding severity.
- Protein C deficiency: Can be inherited or acquired, increasing risk of thrombosis but also associated with bleeding in severe cases.
- Protein S deficiency: Similar to protein C deficiency, with both thrombosis and bleeding risks.
Others
- Von Willebrand disease: Not strictly a factor deficiency, but affects the function of von Willebrand factor, causing easy bruising and mucosal bleeding.
- Disseminated intravascular coagulation (DIC): Not a deficiency, but an abnormal activation of the coagulation cascade leading to excessive clot formation and bleeding.
- Vitamin K deficiency: This essential vitamin is needed for the activation of several clotting factors, and its deficiency can lead to spontaneous bleeding episodes.
General Treatment and Management
Understanding the intricate steps of the cascade is crucial for pinpointing the specific factor responsible for a bleeding disorder. By analyzing the specific stage of the cascade where clotting fails, doctors can diagnose the underlying defect and recommend appropriate treatment. This might involve:
- Replacement therapy: Infusing the missing factor back into the patient’s blood, enabling proper clot formation.
- Medications: Drugs that stimulate the production of certain factors or bypass the defective step in the cascade.
- Blood products: Transfusions with platelets or plasma concentrates can provide temporary support for clotting.
While treatment remains important, understanding the cascade also plays a vital role in preventing unwanted clotting. In conditions like deep vein thrombosis or pulmonary embolism, where clots form within blood vessels, anticoagulant medications come into play. These drugs target specific factors in the cascade, like thrombin or factor Xa, to inhibit their activity and prevent clot formation.
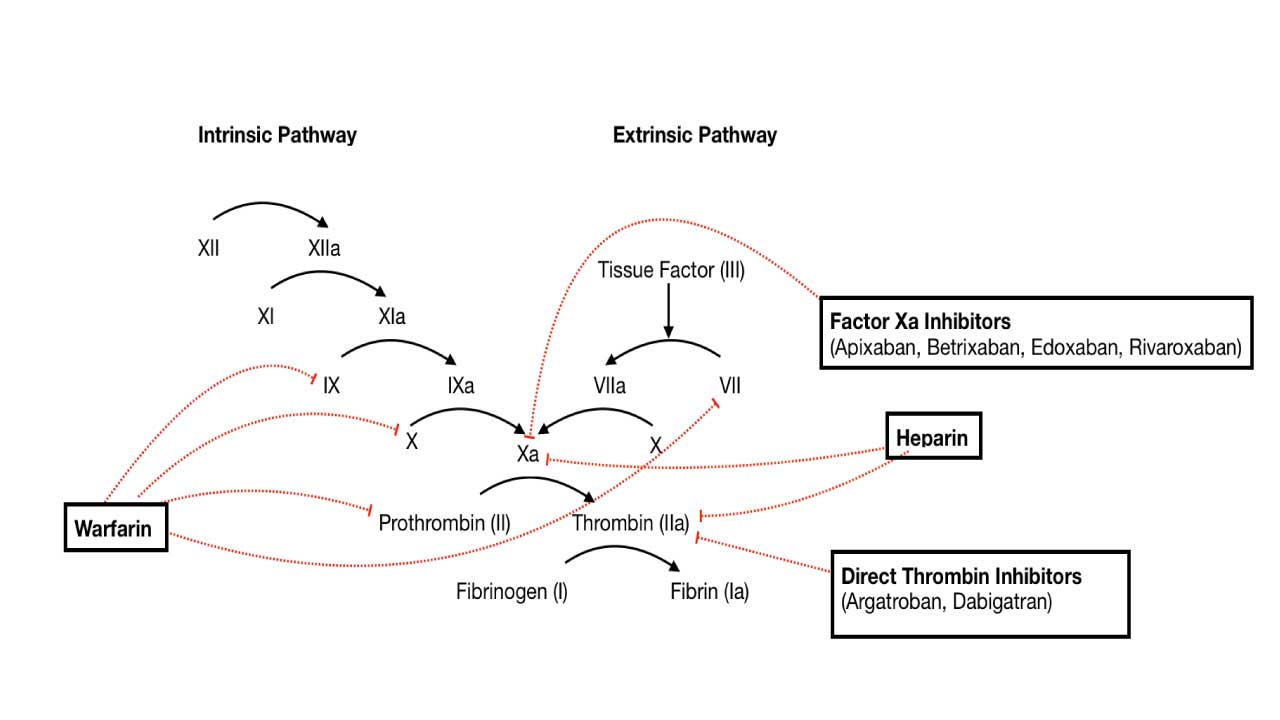
Frequently Asked Questions (FAQs)
What is PT and PTT in the coagulation cascade?
PT (Prothrombin Time) and PTT (Partial Thromboplastin Time) are two essential laboratory tests used to evaluate the coagulation cascade. They help identify potential bleeding disorders or monitor the effectiveness of anticoagulant therapy.
Prothrombin Time (PT)
- Measures the integrity of the extrinsic pathway and the common pathway.
- Evaluates factors VII, X, II (prothrombin), V, and fibrinogen.
- Used to monitor patients on warfarin therapy.
- Often reported as an International Normalized Ratio (INR) to standardize results across laboratories.
Partial Thromboplastin Time (PTT)
- Measures the integrity of the intrinsic pathway and the common pathway.
- Evaluates factors XII, XI, IX, VIII, X, II (prothrombin), V, and fibrinogen.
- Used to monitor patients on heparin therapy.
- Helps diagnose hemophilia A and B.
In essence, PT focuses on the later stages of the coagulation cascade, while PTT assesses the earlier steps. By combining both tests, clinicians can gain a comprehensive understanding of the patient’s clotting ability.
How does warfarin affect the coagulation cascade?
Warfarin is an anticoagulant medication that works by interfering with vitamin K, a crucial cofactor for several proteins involved in the coagulation cascade.
Mechanism of Action
Warfarin inhibits the enzyme vitamin K epoxide reductase, which is responsible for recycling vitamin K after its use in the synthesis of specific clotting factors. By blocking this enzyme, warfarin effectively depletes the body’s supply of active vitamin K.
Impact on Coagulation Factors
Vitamin K is essential for the synthesis of several key clotting factors, including:
- Factors II, VII, IX, and X: These factors are directly involved in the coagulation cascade, leading to thrombin formation and fibrin clot formation.
- Proteins C and S: These are natural anticoagulants that help regulate the clotting process.
By inhibiting vitamin K, warfarin indirectly reduces the levels of these clotting factors, leading to a prolonged prothrombin time (PT) and an increased risk of bleeding.
In essence, warfarin disrupts the normal coagulation cascade by interfering with the production of essential clotting factors, thereby reducing the body’s ability to form clots.
Disclaimer: This article is intended for informational purposes only and is specifically targeted towards medical students. It is not intended to be a substitute for informed professional medical advice, diagnosis, or treatment. While the information presented here is derived from credible medical sources and is believed to be accurate and up-to-date, it is not guaranteed to be complete or error-free. See additional information.
References
- Saba HI, Roberts HR. Hemostasis and Thrombosis: Practical Guidelines in Clinical Management (Wiley Blackwell). 2014.
- DeLoughery TG. Hemostasis and Thrombosis 4th Edition (Springer). 2019.
- Keohane EM, Otto CN, Walenga JM. Rodak’s Hematology 6th Edition (Saunders). 2019.
- Kaushansky K, Levi M. Williams Hematology Hemostasis and Thrombosis (McGraw-Hill). 2017.