Introduction
HPLC, or High Performance Liquid Chromatography, is a powerful tool used to detect hemoglobinopathies. Hemoglobinopathies, a group of genetic disorders that affect the structure or production of hemoglobin, the oxygen-carrying protein in red blood cells, are among the most common single-gene disorders worldwide. These disorders arise from mutations in the genes encoding hemoglobin subunits, leading to alterations in the protein’s structure, function, and stability. Hemoglobinopathies encompass a wide spectrum of conditions, ranging from mild anemia in asymptomatic cases to severe, life-threatening complications.
Hemoglobinopathies are broadly classified into two main categories: thalassemias and structural hemoglobin variants.
- Thalassemias: Thalassemias result from mutations in the genes encoding globin chains, leading to either reduced or absent production of specific globin chains. This imbalance in globin chain synthesis disrupts hemoglobin formation, resulting in anemia, a condition characterized by low red blood cell count or hemoglobin levels.
- Structural hemoglobin variants: Structural hemoglobin variants arise from mutations in the genes encoding globin chains, leading to alterations in the amino acid sequence of the protein. These alterations can affect hemoglobin’s structure, stability, and oxygen-binding affinity, leading to various clinical manifestations.
Among the most prevalent hemoglobinopathies are:
- Sickle cell disease (SCD): SCD is caused by a single mutation in the beta-globin gene, resulting in the production of abnormal hemoglobin S. Hemoglobin S, under low oxygen conditions, can polymerize within red blood cells, causing them to sickle and become rigid, leading to pain, fatigue, and other complications.
- Beta-thalassemia: Beta-thalassemia results from mutations in the beta-globin gene, leading to reduced or absent production of beta-globin chains. The severity of beta-thalassemia depends on the number of mutated genes inherited.
- Alpha-thalassemia: Alpha-thalassemia arises from mutations in the alpha-globin gene, leading to reduced or absent production of alpha-globin chains. Alpha-thalassemia can range from mild, asymptomatic cases to severe, transfusion-dependent anemia.
Diagnosis of hemoglobinopathies typically involves a combination of family history, clinical evaluation, and laboratory tests. Accurate identification of hemoglobin subtypes is essential for diagnosing and managing these conditions. High performance liquid chromatography (HPLC) has emerged as a valuable tool for hemoglobin subtype identification due to its high sensitivity, specificity, and ability to separate and quantify various hemoglobin variants.
Principle of High Performance Liquid Chromatography (HPLC)
High performance liquid chromatography (HPLC) is a versatile and powerful analytical technique widely used in various scientific disciplines, including chemistry, biochemistry, pharmaceutical science, and environmental science. Its ability to separate and quantify complex mixtures of compounds with high sensitivity and resolution has made it an indispensable tool for a wide range of applications.
The Essence of HPLC: Separation and Detection
At the heart of high performance liquid chromatography (HPLC) lies the principle of separation based on the differential distribution of solutes between a stationary phase and a mobile phase. The stationary phase is typically a solid material packed inside a chromatographic column, while the mobile phase is a liquid solvent that flows through the column. As the sample mixture is injected into the column, the solutes interact with the stationary phase and the mobile phase to varying degrees, causing them to travel through the column at different speeds. This separation is achieved based on the interplay of various factors, including the solute’s molecular size, polarity, and affinity for the stationary phase.
The Stationary Phase: The Scaffold for Separation
The stationary phase plays a crucial role in the separation process. It provides a surface for solutes to interact with and influences their distribution between the stationary and mobile phases. Common stationary phases include silica gel, alumina, and bonded phases, which have specific functional groups attached to their surface to enhance selectivity for certain types of solutes.
The Mobile Phase: The Driving Force for Separation
The mobile phase, in the form of a liquid solvent, continuously flows through the chromatographic column, carrying the solutes along its path. The choice of solvent and its composition significantly impact the separation process. The solvent should be compatible with the stationary phase and the solutes to ensure proper interaction and separation.
Detection and Quantification
As solutes elute from the column, they are detected and quantified using a variety of detectors. Common detectors include UV-visible absorbance detectors, which measure the absorbance of light at specific wavelengths, and mass spectrometers, which identify and quantify solutes based on their mass-to-charge ratio.
HPLC Application Hemoglobin Subtype Identification
The ion exchange chromatography for hemoglobin separation relies on the selective interaction between charged groups on the silica-based exchange material (stationary phase) and the charged groups on the hemoglobin molecule (mobile phase). The silica surface is modified with carboxyl groups, imparting a weak cationic charge, enabling the separation of hemoglobin (Hb) molecules based on their charge differences. When a hemolysate containing a mixture of hemoglobins is introduced onto the resin, the elution rate of different hemoglobins is governed by the pH and ionic strength (ion concentration) of the buffered elution solution. The pH and ionic strength of the eluent are carefully controlled to achieve selective elution of different Hb variants.
Separation Mechanism
The separation of hemoglobin variants in high-performance liquid chromatography (HPLC) is primarily driven by two factors:
- Charge: Hemoglobin variants differ in their net charge due to variations in their amino acid sequences. The stationary phase can be designed to selectively interact with hemoglobin variants based on their charge, allowing for their separation.
- Hydrophobicity: Hemoglobin variants also exhibit differences in their hydrophobicity, the tendency of a molecule to repel or attract water. The stationary phase can be tailored to selectively interact with hemoglobin variants based on their hydrophobicity, further enhancing separation.
Detection and Quantification
As hemoglobin variants elute from the column, they are detected using a UV-visible absorbance detector. Hemoglobin absorbs light at specific wavelengths, and the intensity of the absorbed light is proportional to the concentration of the hemoglobin variant. This information is used to quantify the relative proportions of different hemoglobin subtypes in the sample.
Advantages of HPLC for Hemoglobin Subtype Identification
HPLC offers several advantages for hemoglobin subtype identification:
- High Sensitivity: High performance liquid chromatography (HPLC) can detect even small amounts of hemoglobin variants, making it suitable for analyzing samples with low hemoglobin concentrations.
- Specificity: High performance liquid chromatography (HPLC) can distinguish between closely related hemoglobin variants, providing accurate subtype identification.
- Quantification: High performance liquid chromatography (HPLC) allows for the quantification of different hemoglobin variants, providing insights into their relative proportions in the sample.
- Automation: High performance liquid chromatography (HPLC) methods can be automated, reducing manual labor and improving consistency.
VARIANTTM II Beta Thalassemia Short Program (Bio-Rad Laboratories Inc., Hercules, CA, USA)
The VARIANTTM II Beta Thalassemia Short Program employs the principles of ion-exchange high-performance liquid chromatography (HPLC) to accurately identify and quantify hemoglobin variants. This automated method utilizes the VARIANTTM II Sampling Station (VSS) for sample preparation and injection and the VARIANTTM Chromatographic Station (VCS) for separation and detection.
Sample Preparation and Injection
The VSS automates the sample mixing, dilution, and injection process, ensuring precision and consistency. Prepared samples are then injected onto the analytical cartridge for separation and analysis.
Separation and Detection
The VCS employs dual pumps to deliver a precisely controlled gradient of increasing ionic strength to the analytical cartridge. This gradient facilitates the separation of HbA2 and HbF based on their differential ionic interactions with the cartridge material. The separated hemoglobin variants pass through a flow cell equipped with a filter photometer, which measures the absorbance changes at 415 nm. An additional filter at 690 nm compensates for background absorbance.
Data Analysis and Interpretation
The VARIANTTM II CDM (CDM) Software processes the raw data collected from each analysis. To aid in result interpretation, predefined windows have been established for commonly encountered hemoglobins based on their characteristic retention times. For each sample, the CDM generates a sample report and a chromatogram, providing detailed information on the eluted hemoglobin fractions, their retention times, peak areas, and fractional values.
Materials
- EDTA peripheral blood sample
- VARIANTTM Automated HPLC system
- Diluent buffer
- 2 phosphate buffers of different pH and ionic strengths
- HbA2/Hb F calibrator
- Lyphochek® Hemoglobin A2 Control, Bilevel (2 each of 2 levels)
Protocol
- To ensure the accuracy of the subsequent analyses, the HbA2/HbF Calibrator is analyzed at the start of each run to generate calibration factors for both hemoglobin A2 and F. These calibration factors will then be applied to calculate the area percentages for HbA2 and F in all subsequent analyses within the run. Additionally, the Lyphocheck Hemoglobin A2 Control (Levels 1 and 2 from Bio-Rad) is analyzed to verify that the concentration values of HbA2 and HbF remain within acceptable limits.
- A total of 5 uL EDTA blood sample is diluted with a 1 mL diluent buffer and followed by an analysis time of 6.5 minutes per sample.
- For individuals with low hemoglobin readings, they must be pre-diluted in the ratio of 1:100 wash buffer in order to fit the total area within 1,000,000 to 3,000,000 µvolt·second to be considered as valid results.
Interpretation
Peripheral whole blood can be effectively resolved into hemoglobin A, A2/E, F and other haemoglobins variants. This program has two calibrated areas (%), namely peak F and A2, whereby the HbF and HbA2 concentration can be measured.
Manufacturer assigned windows for Bio-Rad VARIANTTM HPLC System
Peak Name | Window (minutes) | Retention Time (minutes) |
F window | 1.00 – 1.30 | 1.15 |
P2 window | 1.30 – 1.60 | 1.45 |
P3 window | 1.60 – 1.90 | 1.75 |
A0 window | 1.90 – 3.30 | 2.60 |
A2 window | 3.30 – 3.90 | 3.60 |
D window | 3.90 – 4.30 | 4.10 |
S window | 4.30 – 4.70 | 4.50 |
C window | 4.90 – 5.30 | 5.10 |
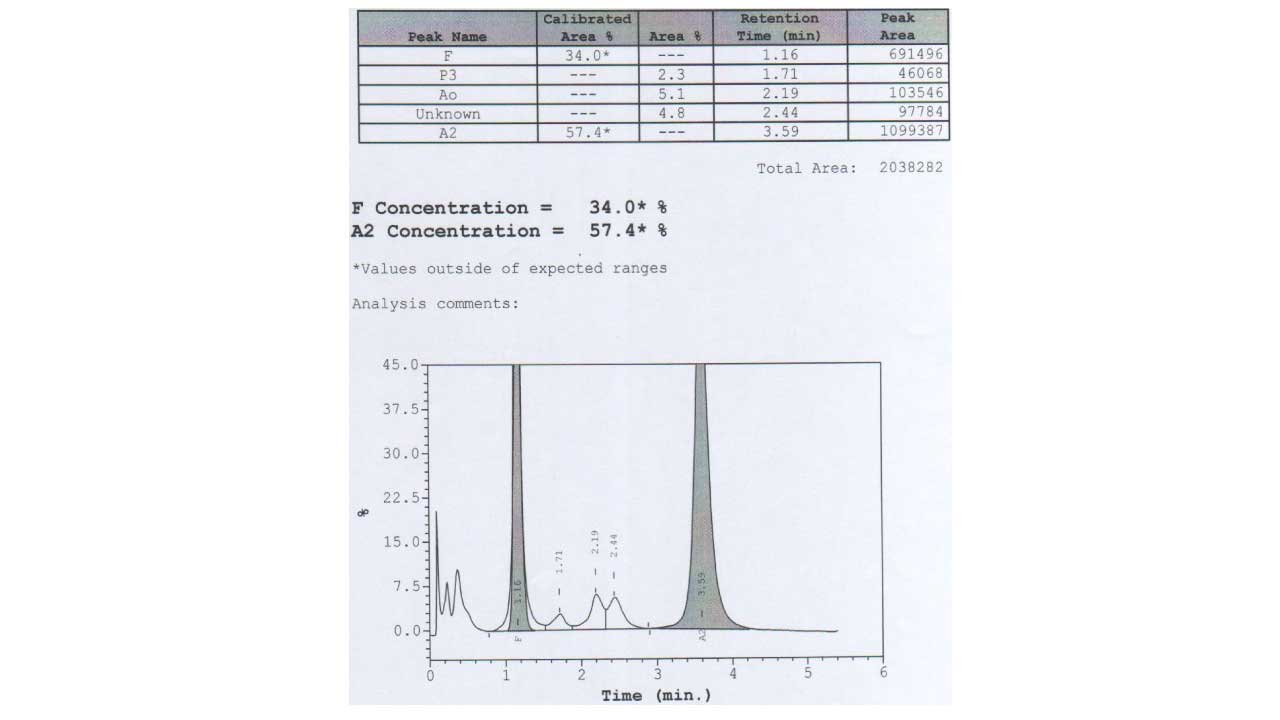
Frequently Asked Questions (FAQs)
What is HPLC best used for?
High-performance liquid chromatography (HPLC) excels at analyzing and separating a wide range of mixtures. Here are some of its most common applications:
1. Quality Control
- Pharmaceuticals: Ensuring the purity and consistency of drugs by identifying and measuring active ingredients and impurities across batches.
- Food and Beverage Industry: Verifying the quality and composition of food and drinks, detecting additives, preservatives, and potential contaminants.
2. Research Applications
- Drug Development: Identifying and characterizing new drug candidates, monitoring their breakdown products in the body.
- Biological Samples: Analyzing proteins, vitamins, and other biomolecules in blood, tissue samples, etc. This can aid in medical diagnosis and research.
- Environmental Analysis: Detecting pollutants like pesticides or herbicides in water and soil samples.
3. Other Applications
- Forensics: Identifying unknown substances in criminal investigations, like analyzing drug samples or explosives.
- Material Science: Characterizing polymers and other materials.
Is HPLC quantitative or qualitative?
High performance liquid chromatography (HPLC) can actually be used for both quantitative and qualitative analysis.
- Quantitative analysis: High performance liquid chromatography (HPLC) is best in measuring the amount of a specific component in a mixture. The detector in the high performance liquid chromatography (HPLC) system generates a signal based on the amount of analyte present. By comparing this signal to a calibration curve created using known standards, scientists can determine the concentration of the target compound in the sample.
- Qualitative analysis: While not its primary strength, high performance liquid chromatography (HPLC) can also be used to identify components in a mixture. This is often done by comparing the retention time (how long it takes a component to travel through the column) of an unknown peak to the retention time of a known standard compound. Additionally, some high performance liquid chromatography (HPLC) systems are coupled with detectors like mass spectrometry (MS) which provide more definitive identification based on the molecule’s mass-to-charge ratio.
High performance liquid chromatography’s (HPLC) strength lies in its versatility. It can handle complex mixtures, separate components based on subtle differences, and provide accurate quantitative data. This makes it a valuable tool across many scientific disciplines.
What are the advantages and disadvantages of HPLC?
HPLC (High Performance Liquid Chromatography) boasts a range of advantages that make it a powerful analytical tool, but it also has limitations to consider.
Advantages
- High Separation Power: High performance liquid chromatography (HPLC) excels at separating complex mixtures into their individual components due to the strong interaction between the stationary phase and the sample. This allows for highly resolved peaks in the chromatogram, making it easier to identify and quantify individual components.
- Sensitivity: Modern high performance liquid chromatography (HPLC) detectors are incredibly sensitive, allowing for detection of very low concentrations of analytes in a sample. This is crucial for applications like drug metabolite analysis or environmental pollutant detection.
- Versatility: High performance liquid chromatography (HPLC) can be applied to a wide range of samples as long as the components are soluble in a liquid solvent. This makes it a valuable tool across various scientific disciplines.
- Quantification: High performance liquid chromatography (HPLC) can be used to accurately measure the amount of a specific component in a mixture. This is achieved by comparing the detector signal of the unknown sample to a calibration curve obtained with known standards.
- Automation: Modern high performance liquid chromatography (HPLC) systems are highly automated, reducing human error and increasing reproducibility of results.
Disadvantages
- Cost: High performance liquid chromatography (HPLC) instruments are expensive to purchase and maintain. The solvents and columns used can also be costly.
- Complexity: Operating and optimizing high-performance liquid chromatography (HPLC) methods can be complex, requiring trained personnel. Troubleshooting issues can also be time-consuming.
- Sample Preparation: High performance liquid chromatography (HPLC) often requires extensive sample preparation steps, such as filtration and dilution, which can be time-consuming and may lead to sample loss.
- Limited Applicability to Non-Soluble Samples: Compounds that are not soluble in a suitable liquid solvent cannot be analyzed using high-performance liquid chromatography (HPLC).
- Time Consumption: While faster than traditional chromatography methods, high performance liquid chromatography (HPLC) analysis times can still vary depending on the complexity of the sample and the desired separation.
Can HPLC determine concentration?
Yes, HPLC (High Performance Liquid Chromatography) can determine concentration.
- Signal and Concentration: The detector in an high performance liquid chromatography (HPLC) system generates a signal that is proportional to the amount of a particular component (analyte) present in the sample. The stronger the signal, the higher the concentration of the analyte.
- Calibration Curve: To quantify the concentration, scientists use a calibration curve. This curve is created by injecting known concentrations of the analyte (standards) and measuring the detector signal for each standard. By plotting the concentration of the standards on the x-axis and the corresponding signal on the y-axis, a linear relationship is established.
- Unknown Sample: Once the calibration curve is established, the high performance liquid chromatography (HPLC) analysis of the unknown sample is performed. The detector signal obtained for the unknown sample is then compared to the calibration curve. This allows scientists to determine the concentration of the analyte in the unknown sample based on its signal strength and the established relationship between signal and concentration.
How does HPLC determine purity?
HPLC (High Performance Liquid Chromatography) is a powerful tool for determining the purity of a sample.
Separation and Peak Areas: High performance liquid chromatography (HPLC) separates the components in a sample based on their interactions with the stationary phase. In a pure sample, you’ll ideally see a single peak in the chromatogram corresponding to the target compound. Any other peaks present would indicate impurities.
Quantification of Peaks: High performance liquid chromatography (HPLC) detectors don’t directly measure purity. However, they can quantify the amount of each component present based on the area under the corresponding peak in the chromatogram.
Purity Calculation: There are two main ways to calculate purity using HPLC data
- Percentage Area Method: This method takes the ratio of the area of the peak corresponding to the target compound (the main peak) to the total area of all peaks in the chromatogram. This ratio is then multiplied by 100% to express the purity as a percentage. A higher percentage indicates a purer sample.
- Normalization: Here, the area of all peaks excluding the target compound (impurities) is summed. This sum is then divided by the total area of all peaks (including the target compound) and multiplied by 100% to give the percentage of impurities. Purity is then 100% minus the percentage of impurities.
Limitations
- Closely Eluting Impurities: If impurities have similar chemical properties to the target compound, they may elute (exit the column) very close together in the chromatogram. This can make it difficult to separate their peaks and accurately quantify them, potentially leading to an overestimation of purity.
- Unknown Impurities: High performance liquid chromatography (HPLC) cannot identify unknown impurities. If a new or unexpected peak appears, it’s challenging to determine its identity without additional analysis techniques like mass spectrometry (MS) coupled with HPLC (HPLC-MS).
What is the most commonly used HPLC?
The most commonly used type of high performance liquid chromatography (HPLC) is Reversed-Phase HPLC (RP-HPLC). There are two main reasons for its widespread use:
- Versatility: Reversed-phase high performance liquid chromatography (HPLC) offers greater compatibility with a wider range of mobile phase solvents. Water is a common component in the mobile phase, making it more environmentally friendly and allowing for analysis of samples that are more soluble in water. Additionally, a variety of organic modifiers like methanol or acetonitrile can be mixed with water to fine-tune the separation for different types of samples.
- Stationary Phase Options: A wider variety of stationary phases are available for reversed-phase high performance liquid chromatography (HPLC) compared to normal-phase HPLC. These stationary phases often have chemically modified surfaces that can interact with specific functional groups on sample components, leading to more selective separations.
Comparison of the two main HPLC types
Feature | Normal-Phase HPLC | Reversed-Phase HPLC |
---|---|---|
Stationary Phase Polarity | More polar | Less polar (non-polar) |
Mobile Phase Polarity | Less polar (non-polar) | More polar (often water-based) |
Separation Principle | More polar components elute slower | More polar components elute faster |
Solvent Compatibility | Less versatile, limited solvent choices | More versatile, wider range of compatible solvents |
Stationary Phase Options | Fewer options | Wider variety of options |
While reversed-phase high performance liquid chromatography (HPLC) is dominant, normal-phase high performance liquid chromatography (HPLC) is still used in specific cases where it offers advantages, such as separating very polar compounds that might not be retained well in reversed-phase systems.
What solvents are compatible with HPLC?
The solvents compatible with high performance liquid chromatography (HPLC) depend on the type of high performance liquid chromatography (HPLC) being used: normal-phase or reversed-phase.
Reversed-Phase high performance liquid chromatography (HPLC) (most common)
- Mobile Phase
- Primary Solvent: Water is a common primary solvent due to its environmental friendliness and ability to dissolve many polar samples.
- Organic Modifiers: Various organic modifiers are mixed with water to fine-tune the separation for different samples. Common choices include:
- Acetonitrile (ACN): Popular choice due to its good miscibility with water, low UV cutoff, and volatility.
- Methanol (MeOH): Another popular modifier, offering good compatibility with water and a lower boiling point than ACN.
- Tetrahydrofuran (THF): Can be used for highly hydrophobic (water-fearing) compounds, but has safety concerns and requires stabilizing agents.
- General Requirements
- High performance liquid chromatography (HPLC) grade solvents are crucial. These are rigorously purified to minimize impurities that could interfere with analysis.
- The solvents should be miscible with each other at the chosen ratios to avoid precipitation in the column.
- Low UV cutoff is important, especially if using a UV detector, to minimize background noise from solvent absorption.
Normal-Phase HPLC (less common)
- Mobile Phase
- Non-polar solvents: Common choices include hexane, heptane, chloroform, and dichloromethane. These are less environmentally friendly than the solvents used in reversed-phase high-performance liquid chromatography (HPLC).
- Alcohols: Sometimes alcohols like methanol or ethanol are used as modifiers to improve solubility of polar solutes.
Why are two solvents normally required in HPLC?
In most cases, two solvents are indeed used in HPLC (High Performance Liquid Chromatography) for the mobile phase, and there are a few key reasons behind this:
- Achieving Optimal Separation: A single solvent often doesn’t provide the necessary range of polarities for effective separation of the components in a mixture. Using two solvents allows you to create a mobile phase with a tunable polarity. By adjusting the ratio of the two solvents, you can fine-tune the strength of the mobile phase and achieve the desired separation for your specific sample.
- Solubility and Elution: One solvent might not be strong enough to dissolve all the components in your sample. A two-solvent system can address this. For example, in reversed-phase high performance liquid chromatography (HPLC), water is often used as one solvent to dissolve polar samples. However, water alone might not be strong enough to elute (push out) some less polar components from the column. Adding an organic modifier like acetonitrile increases the mobile phase’s strength, allowing for better elution of these components.
- Compatibility with Detection: Some detectors used in high performance liquid chromatography (HPLC), like UV detectors, have limitations on the solvents they can work with. A two-solvent system allows you to choose solvents that are compatible with the detector while still achieving the desired separation. For instance, water is a good solvent for UV detection, but using only water might not provide enough selectivity for the separation. Adding an organic modifier can help with separation without significantly affecting UV detection.
Disclaimer: This protocol is intended for informational purposes only and may need to be modified depending on the specific laboratory procedures and patient circumstances. Always consult with a qualified healthcare professional for guidance. See additional information.
References
- George E, Jamal AR, Khalid F, Osman KA. High performance liquid chromatography (HPLC) as a screening tool for classical Beta-thalassaemia trait in malaysia. Malays J Med Sci. 2001 Jul;8(2):40-6. PMID: 22893759; PMCID: PMC3413648.
- PK Gupta, H Kumar, S Kumar, M Jaiprakash. Cation Exchange High Performance Liquid Chromatography for Diagnosis of Haemoglobinopathies. Medical Journal Armed Forces India, 2009;65(1):33-37. https://doi.org/10.1016/S0377-1237(09)80051-8.
- M Dogaru, D Coriu, T Higgins. Comparison of two analytical methods (electrophoresis and HPLC) to detect thalassemias and hemoglobinopathies. Revista Română de Medicină de Laborator 2007; 9(4):39-48.