TL;DR
Sideroblastic anemia is a type of anemia characterized by abnormal iron metabolism within red blood cell precursors. Refractory anemia is defined by the presence of many pathological ring sideroblasts in the bone marrow.
Causes ▾
- Inherited: Mutations in genes involved in heme synthesis (X-linked, autosomal recessive, rare forms).
- Acquired: Alcohol abuse, medications, heavy metal toxicity, myelodysplastic syndromes (MDS), vitamin B6 deficiency (rare), other conditions.
Signs and Symptoms ▾
- Fatigue and weakness
- Pale skin
- Shortness of breath
- Rapid heartbeat (tachycardia)
- Splenomegaly (enlarged spleen) in some cases
- Additional symptoms may depend on the underlying cause (iron overload, alcohol abuse symptoms).
Laboratory Investigations
- CBC: Microcytic or macrocytic anemia, low hemoglobin/hematocrit, normal/low reticulocyte count.
- Peripheral Blood Smear: May reveal anisocytosis, poikilocytosis, basophilic stippling (lead poisoning).
- Bone Marrow Examination with Prussian Blue Stain: Definitive test, presence of numerous ring sideroblasts is diagnostic.
- Iron Studies: Variable results – serum iron may be high/normal, ferritin may be high/normal, transferrin saturation often elevated.
- Additional Tests: Genetic testing (inherited forms), tests for underlying conditions (e.g., alcohol abuse).
Treatment and Management ▾
- Addressing the underlying cause (if possible).
- Supportive therapy: Blood transfusions (severe cases), folic acid supplementation.
- Iron chelation therapy (for iron overload).
- Specific therapies for certain types: Vitamin B6 (XLSA), bone marrow transplant (rarely).
- General management: Regular monitoring, lifestyle modifications.
*Click ▾ for more information
Introduction
Sideroblastic anemia is a type of anemia characterized by abnormal iron metabolism within red blood cell precursors. It belongs to a category called refractory anemia, which means the bone marrow has difficulty producing healthy red blood cells.
The defining feature of sideroblastic anemia is the presence of a high number of pathological ring sideroblasts in the bone marrow. These ring sideroblasts are immature red blood cells with excessive iron deposits in their mitochondria, forming a ring-like structure around the nucleus when visualized under a microscope using a special stain (Prussian blue). This abnormal iron accumulation disrupts the development and function of red blood cells, leading to anemia.
Heme Synthesis and its Importance in Red Blood Cells
Heme, a molecule consisting of iron nestled within a complex organic ring structure (protoporphyrin IX), plays a vital role in red blood cells (RBCs).
Porphyrin synthesis is the process that produces heme. Heme synthesis occurs partly in the mitochondria and partly in the cytosol. The biosynthesis involves an 8-step enzymatic pathway.
Heme biosynthesis begins in the cellular powerhouse, the mitochondria. Here, glycine, an amino acid, joins forces with succinyl-CoA, a molecule derived from the citric acid cycle. This condensation reaction, catalyzed by aminolevulinic acid synthase, forms a crucial intermediate: 5-aminolevulinic acid (ALA).
ALA then takes a trip outside the mitochondria to the cytosol. Here, two ALA molecules come together to form a pyrrole ring compound called porphobilinogen (PBG) with the help of ALA dehydratase (also known as porphobilinogen synthase).
The pathway continues with the assembly of four PBG molecules into hydroxymethylbilane, facilitated by porphobilinogen deaminase. This linear structure needs to cyclize correctly to form uroporphyrinogen III, a process steered by uroporphyrinogen-III synthase. Mistakes at this step can lead to a condition called protoporphyria.
With the correct porphyrin ring (type III) in place, uroporphyrinogen III undergoes side chain modifications by uroporphyrinogen decarboxylase, resulting in coproporphyrinogen III. This newly formed molecule gets transported back into the mitochondria.
Inside the mitochondria, coproporphyrinogen oxidase steps in, removing a carboxyl group from coproporphyrinogen III to create protoporphyrinogen IX. Finally, another round of oxidation by protoporphyrinogen oxidase transforms protoporphyrinogen IX into protoporphyrin IX.
The grand finale involves the insertion of iron into the center of protoporphyrin IX. This crucial step, catalyzed by ferrochelatase, leads to the formation of the much-needed heme molecule.
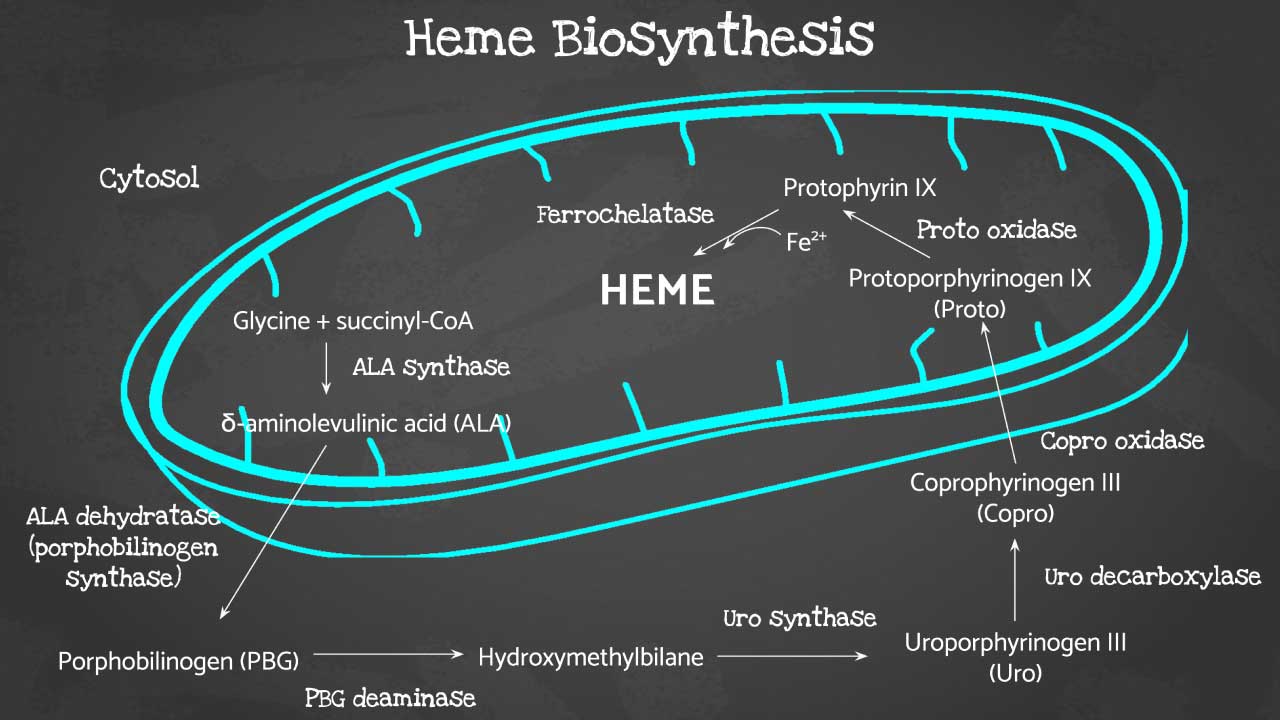
Consequences of Disrupted Heme Synthesis
Disruptions in the heme synthesis pathway can lead to various health problems, including:
- Sideroblastic Anemia: As mentioned earlier, abnormal iron accumulation due to impaired heme synthesis can cause this type of anemia.
- Iron Overload: If excess iron cannot be incorporated into heme, it can accumulate in tissues, leading to organ damage.
- Neurological Issues: Heme precursors can be neurotoxic in some conditions, affecting the nervous system.
Causes of Sideroblastic Anemia
Sideroblastic anemia can be caused by underlying issues that disrupt heme synthesis within red blood cell precursors. These disruptions can be due to genetic mutations (inherited) or acquired factors that affect the process. Here’s a breakdown of the main causes:
Inherited Sideroblastic Anemias
These are caused by mutations in genes encoding enzymes or proteins involved in heme synthesis. The most common types include:
- X-linked Sideroblastic Anemia (XLSA): The most frequent form, caused by mutations in the ABCB7 gene on the X chromosome. This gene plays a role in iron transport within the mitochondria, crucial for heme synthesis.
- Autosomal Recessive Sideroblastic Anemias (ARSA): Mutations in various genes involved in heme synthesis pathways, such as ALAS2 (encoding aminolevulinic acid synthase) or SLC25A38 (involved in iron import). These mutations are inherited from both parents who carry the abnormal gene.
Acquired Sideroblastic Anemias
These develop later in life due to various factors that can disrupt heme synthesis. Common causes include:
- Alcohol Abuse: Chronic alcohol consumption can interfere with iron utilization and heme synthesis.
- Medications: Certain medications, particularly those used for tuberculosis (isoniazid, ethionamide), can have side effects that disrupt heme synthesis.
- Heavy Metal Toxicity: Lead and arsenic poisoning can disrupt enzymes involved in heme synthesis.
- Myelodysplastic Syndromes (MDS): A group of bone marrow disorders affecting blood cell production, including red blood cells. In some cases of MDS, abnormal red blood cell precursors with ring sideroblasts can develop.
Signs and Symptoms
- Fatigue and weakness
- Pale skin
- Shortness of breath
- Rapid heartbeat (tachycardia)
- Splenomegaly (enlarged spleen) in some cases
Laboratory Investigations
Diagnosing sideroblastic anemia involves a combination of laboratory tests to assess red blood cell health, iron status, and the presence of ring sideroblasts, the hallmark feature of this condition.
Complete Blood Count (CBC) with Reticulocyte Count
In sideroblastic anemia, the CBC typically shows:
- Microcytic or macrocytic anemia: In hereditary forms of the anemia, it is usually characterized by hypochromic microcytic anemia.
- Low hemoglobin and hematocrit levels: Reflecting decreased oxygen-carrying capacity.
- Normal or low reticulocyte count: A low count suggests impaired red blood cell production.
Peripheral Blood Smear
In some cases of sideroblastic anemia, the smear might reveal basophilic stippling (bluish granules within red blood cells) – more commonly seen in lead poisoning-induced sideroblastic anemia.
Bone Marrow Examination with Prussian Blue Stain
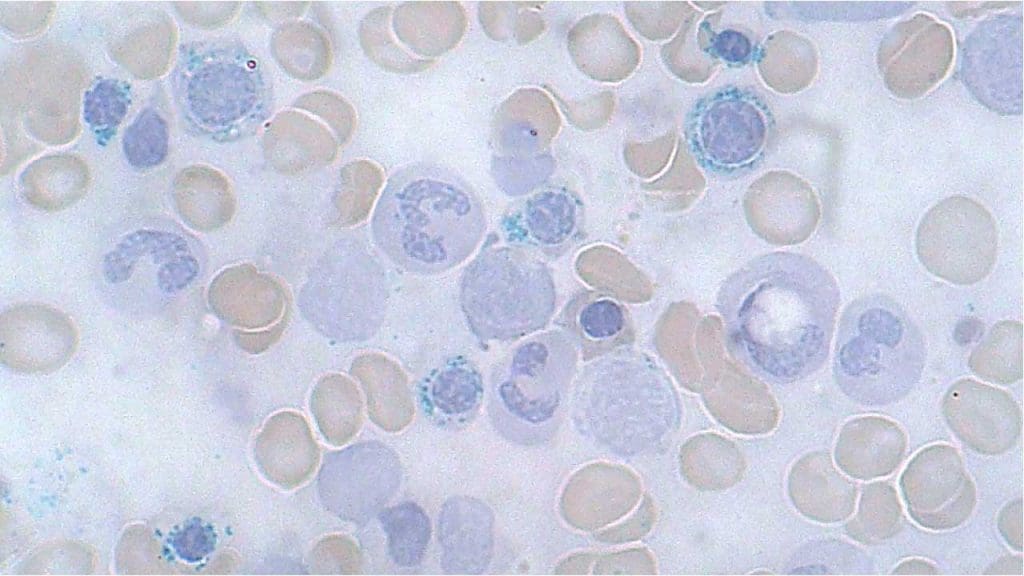
This is the definitive test for diagnosing sideroblastic anemia. A sample of bone marrow is aspirated and examined under a microscope. The crucial step involves staining the sample with Prussian blue, which selectively binds to iron. In sideroblastic anemia, the characteristic finding is the presence of a significant number of ring sideroblasts. These are immature red blood cells with excessive iron deposits arranged in a ring-like structure around the nucleus.
Iron Studies
In sideroblastic anemia, the results can be variable:
- Serum iron levels may be high or normal.
- Serum ferritin levels may be high or normal, depending on iron stores and the cause of the anemia.
- Transferrin saturation is often elevated, indicating excess iron even if total iron levels aren’t necessarily high.
Additional Tests (Depending on Suspected Cause)
- Genetic testing: May be considered to identify mutations associated with inherited forms of sideroblastic anemia.
- Tests for underlying conditions: Depending on the suspected cause (e.g., alcohol abuse, heavy metal poisoning), additional tests might be performed to confirm the underlying condition.
Treatment and Management
The treatment and management of sideroblastic anemia depend on the underlying cause and the severity of the condition.
Addressing the Underlying Cause (if possible)
- Inherited forms: Unfortunately, there’s no cure for inherited sideroblastic anemia. However, treatment focuses on managing symptoms and addressing any complications.
- Acquired forms: If the cause is identified (e.g., alcohol abuse, medication side effects), the first step is eliminating or modifying the triggering factor. This can significantly improve red blood cell production.
Supportive Therapy
- Blood Transfusions: In severe cases with significant anemia and debilitating symptoms, blood transfusions can be used to temporarily increase red blood cell count and improve oxygen delivery. However, frequent transfusions can lead to iron overload, requiring additional management.
- Folic Acid Supplementation: Folic acid deficiency can worsen anemia. Supplementation is often recommended to ensure adequate folate levels.
Iron Chelation Therapy (for Iron Overload)
- In some cases, particularly with acquired sideroblastic anemia, excess iron can accumulate in the body. Iron chelation therapy uses medications to bind iron and facilitate its removal from the body, preventing organ damage.
Specific Therapies
- Vitamin B6 (Pyridoxine) Therapy: X-linked sideroblastic anemia (XLSA) can sometimes respond to pyridoxine supplementation. This can help improve red blood cell production in some individuals.
- Bone Marrow Transplant (Severe Cases): In very severe or life-threatening cases, particularly with inherited forms, bone marrow transplantation might be considered. This is a complex procedure with significant risks and benefits that need careful evaluation.
General Management
- Regular Monitoring: Patients with sideroblastic anemia require regular monitoring of blood counts, iron levels, and overall health to assess treatment response and identify any potential complications.
- Lifestyle Modifications: Maintaining a healthy diet rich in vitamins and minerals can support overall health and red blood cell production. Additionally, managing stress and getting adequate sleep can contribute to well-being.
Overall Prognosis
The prognosis for sideroblastic anemia varies depending on the cause and severity. Inherited forms are lifelong conditions, but with proper management, individuals can maintain a good quality of life. Acquired forms can be more manageable if the underlying cause can be addressed. Early diagnosis and treatment are crucial for optimal outcomes.
Frequently Asked Questions (FAQs)
Why does vitamin B6 deficiency cause sideroblastic anemia?
Vitamin B6 deficiency can contribute to sideroblastic anemia by disrupting a critical step in heme synthesis within red blood cell precursors.
The Role of Vitamin B6 (Pyridoxine) in Heme Synthesis
Vitamin B6 acts as a coenzyme for an enzyme called delta-aminolevulinic acid synthase (ALAS). This enzyme catalyzes the very first step in the heme synthesis pathway, where glycine and succinyl-CoA are condensed to form 5-aminolevulinic acid (ALA). ALA is a key building block for heme.
Impact of B6 Deficiency
When vitamin B6 levels are deficient, the activity of ALAS is compromised. This leads to a shortage of ALA, the essential starting material for heme synthesis. As a consequence, the entire heme production pathway becomes bottlenecked.
Iron Accumulation and Ring Sideroblasts
Even though iron may be available in the cell, it cannot be efficiently incorporated into heme due to the lack of sufficient ALA. This results in the accumulation of excess iron within the mitochondria of red blood cell precursors. These iron deposits form the characteristic ring structures around the nucleus, leading to the development of ring sideroblasts.
Consequences for Red Blood Cells
The abnormal iron accumulation disrupts the normal maturation and function of red blood cells. This impaired production of healthy red blood cells ultimately contributes to sideroblastic anemia.
Why does copper deficiency cause sideroblastic anemia?
Copper deficiency can contribute to sideroblastic anemia by affecting several aspects of iron metabolism and red blood cell development.
Copper’s Role in Heme Synthesis and Iron Utilization
- Enzyme Cofactor: Copper acts as a cofactor for several enzymes crucial in red blood cell development and heme synthesis:
- Cytochrome c oxidase: This enzyme is essential for the electron transport chain in mitochondria, influencing cellular energy production. It also plays a role in iron metabolism within red blood cells.
- Superoxide dismutase: This enzyme helps scavenge free radicals, protecting cells from oxidative damage.
- Hephaestin: This copper-containing ferroxidase enzyme located in the intestinal lining is responsible for oxidizing ferrous iron (Fe2+) to ferric iron (Fe3+). This conversion is essential for iron absorption from the diet.
- Iron Transport: Copper deficiency can impair iron utilization within the body. Hephaestin, as mentioned above, facilitates iron absorption. Additionally, copper might be involved in iron transport within red blood cell precursors.
Impact of Copper Deficiency
- Disrupted Heme Synthesis: When copper levels are low, the activity of cytochrome c oxidase can be hampered. This enzyme dysfunction can indirectly affect heme synthesis, potentially contributing to iron accumulation within red blood cell precursors.
- Impaired Iron Metabolism: Deficient copper can lead to decreased activity of hephaestin, hindering proper iron absorption. Additionally, potential copper-dependent iron transporters within red blood cell precursors might be affected, leading to iron overload within these cells.
- Oxidative Stress: Reduced superoxide dismutase activity due to copper deficiency can lead to increased oxidative stress within red blood cells. This can further disrupt cellular processes and contribute to impaired red blood cell development.
Consequences for Red Blood Cells
Similar to vitamin B6 deficiency, the combined effects of disrupted heme synthesis, impaired iron utilization, and potential oxidative stress can lead to the formation of ring sideroblasts. Excess iron accumulates within red blood cell precursors, affecting their maturation and function. This ultimately contributes to sideroblastic anemia.
How to differentiate between iron deficiency anemia and sideroblastic anemia?
Differentiating between iron deficiency anemia (IDA) and sideroblastic anemia can be crucial for proper diagnosis and treatment.
Here’s a table summarizing the key differentiating points:
Feature | Iron Deficiency Anemia (IDA) | Sideroblastic Anemia |
Serum Iron | Low | High, Normal, or Low (variable) |
Serum Ferritin | Very Low | High or Normal (variable) |
Transferrin Saturation | Low | Elevated |
Bone Marrow Microscopy | Increased red blood cell production, normal morphology | Ring sideroblasts present |
MCV (Red Blood Cell Size) | Microcytic (smaller) | Microcytic or Macrocytic (variable) |
Underlying Cause | Iron deficiency (dietary, blood loss) | Inherited mutations, acquired factors (alcohol, medications) |
How does alcohol cause sideroblastic anemia?
Alcohol abuse can contribute to sideroblastic anemia through several mechanisms that disrupt heme synthesis and iron utilization within red blood cell precursors.
- Direct Toxic Effect on Bone Marrow: Chronic alcohol consumption can directly damage the bone marrow, the factory where red blood cells are produced. This can impair the overall function of red blood cell precursors, leading to decreased production of healthy red blood cells.
- Disrupted Iron Utilization: Alcohol can interfere with the body’s ability to utilize iron effectively for heme synthesis.
- Decreased Hepcidin Production: Hepcidin is a hormone that regulates iron absorption from the gut and its release from storage sites. Alcohol can suppress the production of hepcidin, leading to excessive iron absorption even if the body doesn’t necessarily need it.
- Mitochondrial Dysfunction: Alcohol can disrupt mitochondrial function within red blood cell precursors. Mitochondria are essential for heme synthesis, and their dysfunction can further impair iron utilization for this process.
- Vitamin and Nutrient Deficiencies: Chronic alcohol abuse can lead to deficiencies in various vitamins and nutrients essential for red blood cell production and heme synthesis. Deficiencies in vitamin B6 (pyridoxine), folate, and copper can all contribute to sideroblastic anemia, further exacerbating the problem.
- Oxidative Stress: Alcohol metabolism generates free radicals, leading to oxidative stress within red blood cell precursors. This oxidative stress can damage cellular components and further impair heme synthesis.
Disclaimer: This article is intended for informational purposes only and is specifically targeted towards medical students. It is not intended to be a substitute for informed professional medical advice, diagnosis, or treatment. While the information presented here is derived from credible medical sources and is believed to be accurate and up-to-date, it is not guaranteed to be complete or error-free. See additional information.
References
- Ashorobi D, Chhabra A. Sideroblastic Anemia. [Updated 2023 Jul 17]. In: StatPearls [Internet]. Treasure Island (FL): StatPearls Publishing; 2024 Jan-. Available from: https://www.ncbi.nlm.nih.gov/books/NBK538287/
- Abu-Zeinah, Ghaith, and Maria T DeSancho. “Understanding Sideroblastic Anemia: An Overview of Genetics, Epidemiology, Pathophysiology and Current Therapeutic Options.” Journal of Blood Medicine 11 (2020): 305–18. doi:10.2147/JBM.S232644.
- Bottomley SS, Fleming MD. Sideroblastic anemia: diagnosis and management. Hematol Oncol Clin North Am. 2014 Aug;28(4):653-70, v. doi: 10.1016/j.hoc.2014.04.008. Epub 2014 Jun 2. PMID: 25064706.
- Anemia: Diagnosis and Treatment (Willis, 2016).
- Management of Anemia: A Comprehensive Guide for Clinicians (Provenzano et al., 2018)
- Goldberg S, Hoffman J. Clinical Hematology Made Ridiculously Simple, 1st Edition: An Incredibly Easy Way to Learn for Medical, Nursing, PA Students, and General Practitioners (MedMaster Medical Books). 2021.